Prioritize...
Upon completing this page, you should be able to compare and contrast the basic structure and evolution of tropical and mid-latitude cyclones. Specifically, you should be able to discuss differences in vertical motion over the centers of mid-latitude cyclones and hurricanes, and the implications of these differences in terms of temperatures, relative humidity, and surface pressure. You should also be be able to define key parts of a hurricane's structure (such as eye, eyewall, spiral bands, and secondary circulation). Finally, you should leave this page being able to summarize the basic feedback process that causes tropical cyclones to intensify.
Read...
With the great potential for loss of life and property posed by tropical cyclones, they certainly garner great attention from weather forecasters and the public at large. But, why do powerful tropical cyclones more frequently steal national and international headlines, while mid-latitude cyclones rarely do? The first reason is likely that mid-latitude cyclones are more numerous. Hundreds of them trek across the globe each year. Meanwhile, only about 80 tropical cyclones develop each year.
Secondly, a tropical cyclone can attain a much greater intensity in terms of both sea-level pressure and wind speed (some even call hurricanes the "kings" of all low-pressure systems). For example, the most intense tropical cyclones can have sea-level pressures below 900 mb. Typhoon Tip (1979) had the all-time lowest at 870 mb, but other storms such as Hurricane Wilma (2005) and Super Typhoon Haiyan (2013) have had central pressures below 900 mb. On the other hand, the sea-level pressure at the center of a mid-latitude cyclone rarely drops below 950 mb. For example, the Superstorm of 1993 (aka the "Storm of the Century"), had a central pressure of 963 mb at its peak.
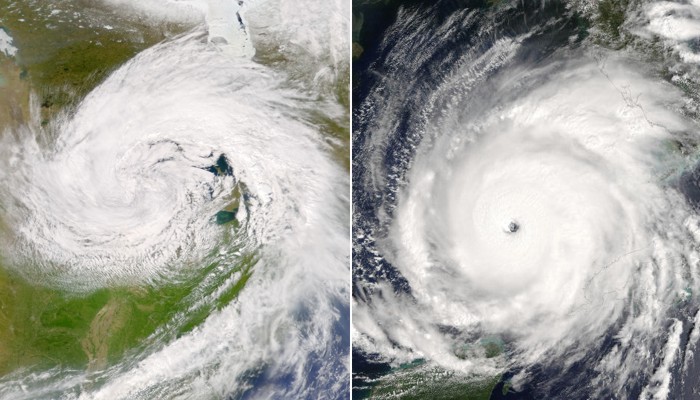
With these observations in mind, a natural question might be, "Why do strong tropical cyclones often attain sea-level pressures that are notably lower than those associated with mid-latitude cyclones?" While both types of cyclones are low-pressure systems, the answer to that question can found by examining the differences in structure and strengthening mechanisms characteristic of each type of low-pressure system.
For starters, recall that mid-latitude cyclones undergo the process of self-development. This process requires the cyclone to develop in a region of strong horizontal temperature gradients (a baroclinic zone, or front) and under a region of strong upper-level divergence. In short, divergence downwind of a 500-mb shortwave trough reduces the weight of air columns, forming an area of low pressure at the surface, around which winds rotate counterclockwise (in the Northern Hemisphere). Decreasing surface pressures result in a stronger pressure gradient force, which causes faster winds. As winds around the cyclone increase, cold-air advection southwest of the low increases, causing 500-mb heights to fall and the 500-mb trough and vorticity maximum to strengthen. In turn, upper-air divergence increases over the center of the low, causing surface pressures to further decrease and surface winds to increase further. This positive feedback loop continues uninterrupted until the late stages of occlusion, when the low moves back into the cold air (away from the baroclinic zone) and upper-level divergence over the low weakens (the low starts to "fill" -- surface pressure rises).
In a nutshell, the magnitude of the divergence aloft (which is greater than the magnitude of the convergence at lower altitudes) drives the intensity of the mid-latitude cyclone. Also note, however, that the divergence aloft along with low-level convergence drives upward motion over the center of the low. You'll occasionally read or hear explanations that suggest that rising causes lower surface pressures, but that's just not true. In fact, just the opposite is true. Rising air actually works against the overall reduction in surface pressure.
Recall that rising air cools via expansion, and once clouds and precipitation develop, can also yield evaporational cooling (assuming the atmosphere is not already at saturation). In turn, cooling by forced ascent increases the mean density in the column of air that extends from the ground to the tropopause (low-level convergence and upper-level divergence are still at work). Assuming a nearly hydrostatic atmosphere (in which the force of gravity is balanced by the upward pressure gradient force), this increase in mean column density serves to add column weight. During the development stage of a mid-latitude cyclone, dominant weight-loss processes, such as net column divergence and warm advection near 200 mb overwhelmingly offset the tendency for air columns to gain weight from adiabatic and moist adiabatic cooling. But my point should now be clear: Rising air tends to make surface pressures higher, not lower. In other words, rising air actually works against the deepening of a mid-latitude cyclone; it serves as a "check and balance" on the overall intensity of the system.
Strong tropical cyclones, on the other hand, don't have this "check and balance" over their centers. Indeed, the predominant vertical motion over the center of a hurricane is downward. It's that downward motion that creates the eye of the storm, as shown in the visible satellite image of Hurricane Isabel from 1404Z on September 11, 2003 (below).
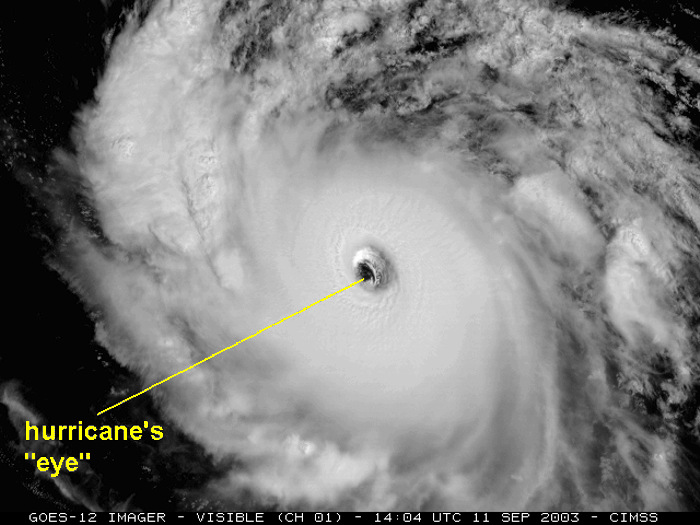
For the record, the eye is a roughly circular, fair-weather zone at the center of a hurricane. By "fair weather", I mean that little or no precipitation occurs in the eye and an observer looking upward in the eye can often see some blue sky or stars. The diameter of the typical eye ranges from approximately 30 to 60 kilometers (about 16 to 32 nautical miles across), but eye diameters as small as four kilometers (approximately two nautical miles) and as large as 200 kilometers (approximately 110 nautical miles) have been observed. For the record, Hurricane Wilma's "pinhole eye" was the smallest recollected by forecasters at the National Hurricane Center (two nautical miles) as the storm deepened to 882 mb (the lowest on record in the Atlantic Basin).
The "fair weather" in the eye can largely be attributed to the sinking air over the center of the storm. The downward motion in the eye is only on the order of a few centimeters per second, which suggests that the central core of strong tropical cyclones is approximately hydrostatic. Given that the compressional warming in the eye decreases the mean density of the central column of air in the eye (and thus its weight), we can deduce that subsidence contributes to the low central pressures observed in hurricanes. Of course, as the sinking air warms, relative humidity decreases within the sinking parcels, which promotes the clearing observed within the eye.
I should point out however, that the air does not uniformly sink within the eye of a hurricane. Dropwindsonde observations taken from the eye of a hurricane often reveal an inversion at an altitude of about one to three kilometers. To see what I mean, check out the representative temperature and dew-point soundings retrieved from dropwindsonde measurements in the eye of Hurricane Jimena (1991). The subsidence inversion near 850 mb is the telltale sign of downward motion in the eye of a hurricane, but the presence of this inversion means that air does not sink all the way to the ocean surface. The fact that air does not sink all the way to the surface explains why low clouds frequently exist in the eyes of hurricanes (although skies may not be completely overcast).
Regardless of the fact that air does not uniformly sink throughout the entire eye, the compressional warming associated with the subsidence in the eye is one contributor to the "warm core" of a hurricane. Meanwhile, deep, moist convection outside of the eye (in the eyewall--the partial or complete ring of powerful thunderstorms around the eye, and spiral bands--relatively long and thin bands of convective rains) also contributes to the warm core.
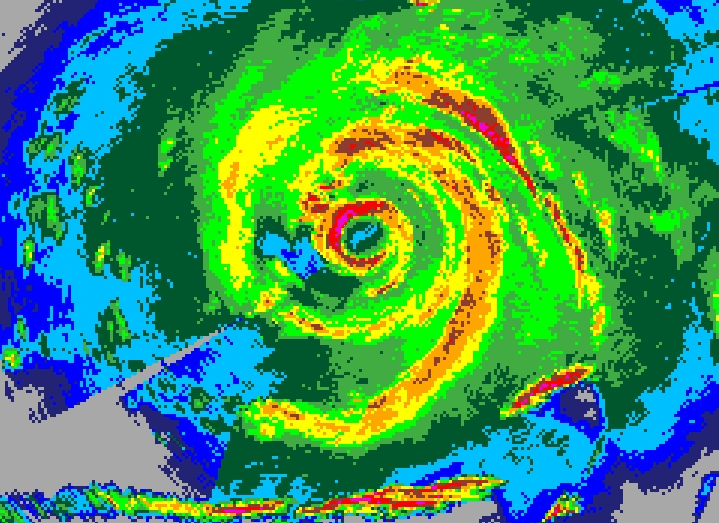
The image above gives you a "bird's-eye" view of the basic structure of a hurricane on radar. Note the spiral bands (yellow, orange, and red shadings) curving in toward the center of the storm, and the eyewall almost completely encircling the much lower reflectivity values (dark green and blue) in the eye. How does the deep, moist convection in the eyewall and spiral bands contribute to the warm core of the storm? Simply put, the air parcels rising in thunderstorm updrafts are initially very warm and moist (due to evaporation from warm tropical seas). As these parcels rise in thunderstorm updrafts, huge amounts of latent heat of condensation are released. Yes, air parcels cool as they rise, but the release of latent heat keeps them warmer than they otherwise would be, which keeps the air within a hurricane warmer than air at the same altitudes outside of the influence of the hurricane. Weaker tropical cyclones are also warm core systems because of the release of abundant latent heat (even though weaker systems don't have eyes--there's no organized compressional warming in the center of the storm).
All in all, within a strong tropical cyclone, the warm core generated by latent heat release and compressional warming can be quite substantial. For example, check out the cross-section of satellite-detected temperature anomalies from Super Typhoon Haiyan at 1726Z on November 7, 2013 (below).
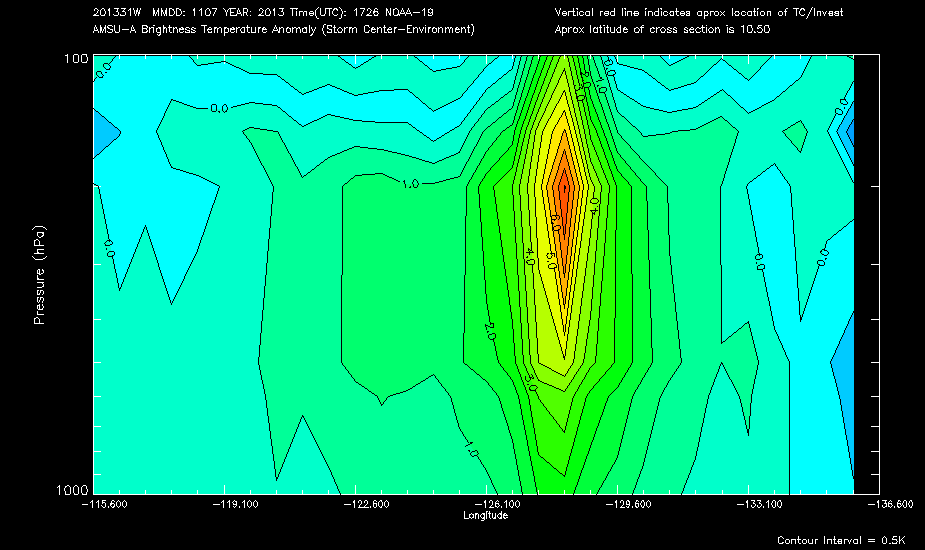
The core of the warm anomaly approximately coincides with the eye of Haiyan, and at its peak in the middle and upper troposphere, temperatures were as much as 7 degrees Celsius greater than the environment surrounding the storm. Outside of the eye, the warm anomaly is weaker, but still spans hundreds of miles across the storm. Given the maximized warm core near the center of the storm, it becomes clear that hurricanes create large horizontal temperature gradients internally (especially at the interface of the eye and eyewall) during their development, even though they initially form in the weak horizontal temperature gradients that characterize the tropics. As you've learned, mid-latitude cyclones are just the opposite: They form in areas with large horizontal temperature gradients, and their circulations ultimately act to reduce horizontal temperature gradients over time.
Sustaining Tropical Cyclones
Now that we've established a key difference between tropical cyclones (which have a warm core) and mid-latitude cyclones (which do not, since they are characterized by rising motion over their centers and typically lack deep, moist convection near their cores), let's turn our attention to another key factor in the intensification of both mid-latitude and tropical cyclones--divergence aloft. You're already familiar with the role of divergence aloft in mid-latitude cyclones, supplied primarily by 500-mb shortwave troughs and 300-mb jet streaks, but divergence aloft plays an important role in tropical cyclones, too.
In order to help you visualize divergence aloft in tropical cyclones, allow me to introduce the secondary circulation of a tropical cyclone. As the name implies, tropical cyclones have two distinct circulations. The primary circulation, as you might expect, refers to rotation of air around the center of the storm. But, there's another circulation going on at the same time. In a basic sense, low-level air flows in toward the center of the storm, rises in thunderstorms within the eyewall and spiral bands, and flows (mostly) outward aloft, sinking around the periphery of the storm. This general circulation (in at the bottom of the storm, up, out at the top, and down around the storm's periphery) is the secondary circulation. To visualize this "in, up, and, out" process in the context of a strengthening hurricane, check out the slideshow animation below.
The divergence aloft in a healthy tropical cyclone acts to further reduce surface pressure by removing mass from air columns near the center of the storm. Ultimately, hurricanes intensify as a result of a positive feedback loop, albeit a completely different one than the self development process for mid-latitude cyclones. One of the salient features in the positive feedback loop for hurricanes is "scale interaction." In a nutshell, processes on the spatial scale of convection (thunderstorms, for example) work to amplify changes on a larger spatial scale (such as lowering surface air pressure in the eye of a hurricane). In turn, amplification on the larger spatial scale amplifies convection (thunderstorms), and the feedback loop is off to the races.
We'll delve much deeper into the details later in the course, but here are the basics of the feedback: As eye-wall thunderstorms mushroom upwards and intensify, the magnitude of the secondary circulation (and divergence aloft) becomes greater, as does subsidence and compressional warming in the eye. This all sets the stage for negative pressure tendencies near the ocean surface (surface pressure decreases with time), which draws more low-level air inward to rise in thunderstorm updrafts, and the cycle continues. The key to maintaining the whole process is sustaining organized deep convection around the core of the storm.
As you now know, tropical cyclones operate quite a bit differently from mid-latitude cyclone, so make sure that you understand the main contrasts between the two types of storms. To help you keep track of the major differences, below is a quick summary, highlighting the key differences between mid-latitude and tropical cyclones.
Key Differences Between Mid-Latitude and Tropical Cyclones
- Mid-latitude cyclones form in environments with strong horizontal temperature gradients, while tropical cyclones form in environments with weak horizontal temperature gradients (but they create strong horizontal temperature gradients internally).
- Air rises over the center of a mid-latitude cyclone, and thus, cools, which works against falling surface pressures. Over the centers of strong tropical cyclones, however, air sinks and warms via compression, which helps surface pressures decrease.
- The release of latent heat from deep, moist convection, and compressional warming from subsidence causes tropical cyclones to have a warm core. Mid-latitude cyclones, on the other hand, lack a warm core.
- Mid-latitude cyclones rely on divergence aloft to drive decreases in surface pressure. Low surface pressures in tropical cyclones, on the other hand, result from significant contributions from the warm core of the storm (low column density) and divergence aloft via the secondary circulation.
By now, I hope you're beginning to appreciate the differences between the mid-latitudes and the tropics. But, we're not done quite yet. Even the tools that tropical forecasters use are different! We'll start with map projections next. You'll quickly see that the map projections commonly used in the mid-latitudes don't work so well in the tropics!
Explore Further...
Mid-Latitude Cyclones with Eyes?
The centers of mid-latitude cyclones are typically quite cloudy due to the upward motion that occurs there. However, some mid-latitude cyclones (particularly those over the oceans), actually exhibit "eye-like" features during their mature phases. Such features occasionally become apparent when intense mid-latitude cyclones spin-up off the East Coastand aren't actually true "eyes" like those in tropical cyclones. Instead, these cloud-free regions in the center of a mid-latitude cyclone are referred to as "warm air seclusions." For example, an intense mid-latitude low off the coast of Long Island, NY, developed an eye-like, warm air seclusion at 15Z on April 16, 2007 (check out the 1515Z visible satellite image below).
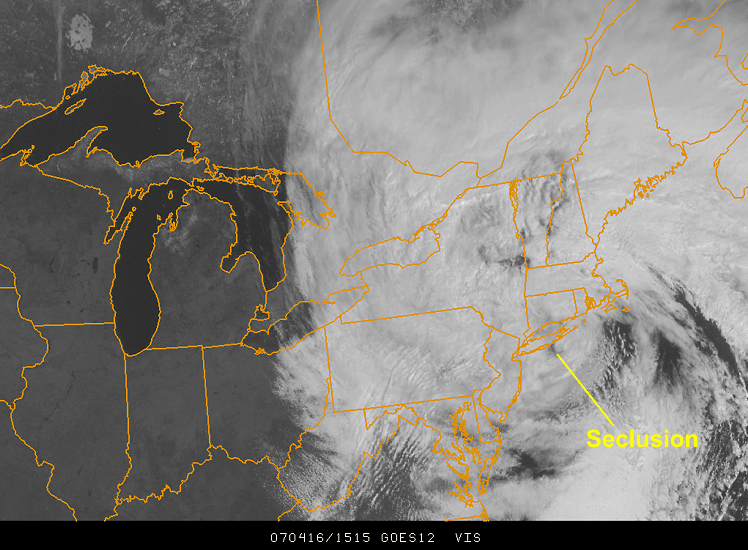
Note that unlike tropical cyclones, no thunderstorms were present around the center of this eye-like feature (check out the 1515Z enhanced infrared image for confirmation -- high cloud-tops indicative of deep convection were certainly lacking). While the details of the formation of such features are well beyond the scope of this course, in a nutshell, air wraps cyclonically around the western flank of the low and traps warm air at the center of circulation, creating a warm air seclusion. The cyclone model, which describes the evolution of these types of cyclones, is called the Shapiro-Keyser Cyclone Model, and it differs somewhat from the classic "Norwegian" cyclone model you're familiar with. If you're interested in the Shapiro-Keyser Cyclone Model and warm air seclusions, here's one of the digestible research papers on this topic. Enjoy!
Can cyclones ever change type?
In order to thrive, tropical cyclones require organized thunderstorms around their centers. In contrast, mid-latitude cyclones require large horizontal temperature contrasts in order to intensify. With these contrasting characteristics in mind, you might assume that tropical cyclones can never crossover into the realm of mid-latitude cyclones, but that's not really true.
As tropical cyclones move poleward, they inevitably enter an environment where there are horizontal temperature gradients. Before dissipating, a tropical cyclone sometimes becomes "extratropical" or "post-tropical," transitioning from a system with thunderstorms around its center to a mid-latitude low-pressure system that derives its energy from synoptic-scale temperature gradients.
A good example of an "extratropical transition" can be seen with Hurricane Noel. Early on November 2, 2007, Hurricane Noel started to move poleward off the coast of Florida. To gain a sense of the overall weather pattern, check out the 06Z surface analysis. Noel's position is marked by the hurricane icon and note the cold front coming off the East Coast. On the 0615Z enhanced water vapor image, it's easy to see the high cloud tops and high concentrations of water vapor in the upper troposphere focused around Noel's center. As Noel advanced north-northeastward (check out Noel's track) toward the cold front later that night, the tropical cyclone became post-tropical as it became embedded in the temperature gradients associated with the front. The 09Z surface analysis on November 3, 2007, indicates the remnants of Noel (marked by the red "L") on the verge of merging with the front.
Another clue to Noel's post-tropical transition is the comma-shaped configuration of the high cloud tops on this enhanced water vapor image at 1515Z the next morning (November 3, 2007). This satellite presentation is consistent with the classic conceptual model of mid-latitude cyclones that you've learned. The National Hurricane Center noted Noel's transition in its last Noel advisory (5 P.M. EDT on November 2).
For the record, "tropical transitions" can occur, too, in which non-tropical cyclones change into tropical cyclones. Often, such cyclones simultaneously exhibit characteristics of both mid-latitude and tropical cyclones for a time (and are called "subtropical cyclones"), but we'll touch on these topics later in the course.