
6.3 Water treatment systems
Water treatment technologies are designed to eliminate harmful effects of pollutants and natural substances to human health and environment. Within the blended water cycle (considered on page 6.1), these technologies are often placed at the transitions between the environment and human sphere to adapt the water quality.
For example, when water passes from the environmental source to the human consumption system, there is a possible risk to human health from some natural bacteria, chemical elements. Hence, natural water (from either surface or underground reservoir) needs to be purified to a certain standard. On the other end of the system, the water containing waste or substances resulting from the domestic, agricultural, or industrial activity must be cleaned before returning to the environmental pathways. If this is not done, harmful effects of concentrated pollutants can cause significant disturbance to the natural water ecology and escalate damage to both ecosystem and society in the long run. Some common effects of wastewater pollution include eutrophication (biological nutrient pollution; for example, releasing access of nitrogen and phosphorus —"overfeeding ecosystem"); oxygen depletion (due to oxidation of organic compounds); odor and aesthetic damage; proliferation of harmful bacteria, viruses, fungi in drinking water supply.
Centralized water treatment
Centralized water treatment approach implies treating large amounts of water at large rates in a "central" location and distributing that water via networks of pipelines, channels, and intermediate reservoirs. Centralized water treatment is largely implemented and maintained in major urban areas and in most parts of the developed world. Probably most of us primarily use the centralized treatment in our lives (maybe except some travel circumstances).
A couple of videos below describe large-scale water treatment systems that are designed to remove undesired contaminants from water.
This first video shows an example of how water is treated during its transfer from the environmental source to the drinking water supply:
Video: Water Treatment Plant Tour (5:26)
Welcome to our tour of Winnipeg's drinking water supply and treatment system. We use leading edge technology to treat the water as it passes through many stages, including coagulation, flocculation, dissolved air flotation, filtration, and three types of disinfection. Our system protects public health by virtually eliminating the risk of waterborne disease and reducing the level of harmful disinfection byproducts. It also improves the taste, odor, and appearance of our tap water.
Shoal Lake has been our source of water since 1919. It's located 136 kilometers from Winnipeg at the Manitoba-Ontario border and is approximately 92 meters higher than Winnipeg. Water flows downhill from Shoal Lake through an aqueduct into four large reservoirs next to the treatment plant. The aqueduct is a large concrete pipe that carries up to 386 million litres of water per day to the reservoirs. The reservoirs hold up to 8.8 billion litres of water which on average is enough to supply Winnipeg for about 30 days.
Water flows from the reservoirs through two large pipes into powerful pumps that move the water into the plant for treatment. This $300 million state of the art facility features a highly automated system that monitors and controls a wide variety of instruments, mechanical equipment, and electrical equipment, including 40 processors, 140 pumps, 2,300 valves, and 1,400 instruments. It can treat up to 400 million litres of water per day.
In this first step, we add sulphuric acid and ferric chloride as the water flows into the plant. Sulphuric acid lowers the pH of the water and makes this stage of treatment more efficient. Ferric chloride, a coagulant, causes the naturally occurring particles of organic material in the water to attract each other. The water enters flocculation basins where large mixers slowly stir the water, causing the particles to collide and stick together, creating large clumps. Now that large clumps have been formed in the water, the next step is to remove them. We do this in dissolved air floatation tanks. We inject a stream of water, supersaturated with air, into the flocculated water which is flowing into the bottom of the tanks. Tiny air bubbles are released into the water and carry the clumps to the top of the tanks. Skimmers remove the floating clumps from the surface of the water. The clumps are pumped to an area for further processing. The treated water is collected from the bottom of the tanks. The water flows from the dissolved air floatation tanks to chambers where ozone is added. This step called ozonation does three things. It provides the first level of disinfection by destroying most of the harmful bacteria, it improves the filter performance in the next treatment stage, and it improves the taste and odor of the water. We make ozone by applying electricity to liquid oxygen. At the end of the ozone chambers, we add sodium bisulphite to the ozonated water to remove any leftover ozone.
The water now flows into filter tanks containing biologically activated carbon. The filters remove any remaining small particles in the water, including parasites. Good bacteria growing on the filters remove some of the remaining dissolved organic material. This reduces the level of disinfection byproducts in the water. We clean the filters regularly by pumping air and water backwards through them. The backwashed water is pumped to an area for additional processing. The filtered water flows into the chlorine contact chamber for a second round of disinfection. We add chlorine to kill viruses and bacteria such as E. coli that might be in the water. Chlorine is the most widely used drinking water disinfectant in North America and has been used for more than 100 years. We then add sodium hydroxide to bring back the pH of the water close to the original level. The water flows from the chlorine contact chamber into an underground reservoir called the clearwell. We disinfect the water once again using ultraviolet, or UV light. We pump the water through six stainless steel chambers, each containing nine ultraviolet lamps. The lamps are similar to fluorescent bulbs. With only seconds of exposure, the UV light rays penetrate any remaining parasites such as Cryptosporidium, or Giardia. The UV light renders these parasites harmless. After UV disinfection, the water flows into two large pipes where we add fluoride to help prevent tooth decay. We also add orthophosphate to form a protective coating inside water pipes. This coating helps reduce corrosion that may add lead to tap water. The two large pipes carry the treated water to our three reservoirs and pumping stations in the city. We add chlorine again as the water is pumped into the distribution system to ensure that the water remains disinfected until it reaches your tap.
The final stage of treatment is to deal with the materials removed from the water by the treatment processes. The backwash water from the filters is allowed to stand until the solid materials settle. We then send the clean water back to the beginning of the treatment process. We pump the settled out material from the filters and the clumps of particles from the dissolved air flotation process to outdoor settling ponds next to the treatment plant. The pond contents go through natural freeze thaw cycles which separate the liquid and solids. We pump the liquid into Winnipeg's sewer system for wastewater treatment and take the solids to the landfill for disposal.
We test our water each step of the way, from shoal lake to your tap. Our testing program includes over 150 different tests at more than 130 different locations throughout the year. Because water quality is so important, we do more testing than the provincial government requires. Our certified professional team is dedicated to ensuring that our community enjoys safe, high quality drinking water that meets provincial regulatory requirements, and falls well within Health Canada guidelines.
We hope that you've enjoyed this tour of our drinking water supply and treatment system.
This second video illustrates the treatment of the wastewater generated by human activity before it is returned to the environment:
Video: Barry's Wastewater Treatment Tour (5:26)
Have you ever wondered what happens to the water that flows down drains and toilets in your home? Hi. I'm Barry. And today I'm going to show you how wastewater gets treated. The journey begins here in your community. When it rains, snows, or when you wash your car in your driveway, the water runoff is known as stormwater. It flows into these storm drains that you see in your neighborhood streets. Storm drains connect to streams and creeks and eventually lead to Lake Ontario. But something different happens to the water that you use in your home. All the water that goes down drains and toilets in your home is known as wastewater. It's full of food, soap, waste, and more. Wastewater never goes straight into the lake or river. From your basement, wastewater flows into underground pipes that follow the natural slope of the land. So, most of the time, wastewater just flows downhill to the wastewater treatment facility. Now let's go see how we treat it. All of the wastewater from thousands of homes and buildings across Mississauga, Brampton, and parts of Caledon arrives here. It's collected in six big underground pipes directly underneath me. Each pipe is six feet in diameter.
Now let's go see the first step in wastewater treatment. The wastewater flows through these moving screens. The screens trap materials that should never be flushed down your toilet, things like plastics, rags, and dental floss. Everything the screens trap ends up on these conveyors. The conveyors bring all the garbage into large bins in the building next door. The garbage from the conveyors is collected here. When these bins are full, trucks take it away to landfill. Next, let's see where the wastewater goes. After the screening, the wastewater travels into underground grit chambers. Each of the chambers is round and spins wastewater, kind of like swirling water in a cup. As it spins, sand and small rocks are separated, move to the center of the chambers, and are removed. Now that the solid particles and garbage have been removed from the wastewater, it flows into settling tanks, like this one. We call these settling tanks because the wastewater is given time to settle. At the top of the tank, scum floats and is made up of grease, fats, and oils. At the bottom of the tank, sludge sinks and is made up of heavier materials, mainly human waste. Over here, we have an empty settling tank, so you can see how deep it is.
So now we have sunken sludge and floating scum. What happens next? See this large machine across the tank? We call this the bridge. But it doesn't stay in one spot like a regular bridge. The bridge slowly travels the length of the tank. As it moves, it scrapes the sludge along the bottom and skims the scum along the top. It takes 30 minutes for the bridge to travel all the way across the tank. Watch. I'll show you. The sludge and the scum are pushed to the end of the tank where it's collected. The sludge, under water, we can't see. But, at the surface, we can see the scum collecting here. This is a continuous process. The bridge will lift, go back to the far end of the tank, and start over.
Now the journey splits in two. First, we'll see where the sludge and scum go. Then we'll come back and see what happens to the wastewater. The sludge and the scum from the settling tanks, now referred to as biosolids, are pumped into these machines called centrifuges. A centrifuge spins biosolids super fast, like the spin cycle of a washing ma the end of the tank where it's collected. The sludge, under water, we can't see. But, at the surface, we can see the scum collecting here. This is a continuous process. The bridge will lift, go back to the far end of the tank, and start over. Now the journey splits in two. First, we'll see where the sludge and scum go. Then we'll come back and see what happens to the wastewater. The sludge and the scum from the settling tanks, now referred to as biosolids, are pumped into these machines called centrifuges. A centrifuge spins biosolids super fast, like the spin cycle of a washing machine, and remove excess water. Now, getting rid of biosolids, that's when things really heat up. This is one of four biosolids incinerators. And it heats up to over 840 degrees Celsius. The biosolids enter the incinerator with the consistency of cake batter. The incinerator then heats up the biosolids until they turn into ash. For every 10 tons of biosolids, we end up with only one ton of ash.
Now let's go see where the ash ends up. This is a lagoon where the ash is stored. The water is red because of the iron minerals that we added to eliminate phosphates. This is just a little ways away from the incinerators over there. So we add water to the ash to make it easier to pump. And that's how biosolids have turned to ash. Let's go back to where our journey split in two and find out what happens to wastewater from the settling tanks. With the biosolids removed from the wastewater, something interesting happens. Wastewater flows into these aeration tanks. Aeration is a process of pumping oxygen into water. Do you see all those bubbles? That's the oxygen. Aeration allows good bacteria to eat the sludge that didn't sink and the scum that didn't float from the settling tanks. This process is what makes wastewater clear, but it's not clean yet. These are the clarifying tanks. Remember the good bacteria that ate everything to make the water clear? They now sink to the bottom of the tanks and are removed. The water at the top of the tank is now clean, and it will be returned to Lake Ontario. Along the way, we add chlorine as a final disinfectant. It is now time for us to return the treated wastewater back to Lake Ontario. An underwater pipe stretches just over two kilometers out and lies at the bottom of the lake. Before we return the treated wastewater, we remove any excess chlorine.
Now let's go take a look at the control room. Here's where we monitor all the processes we talked about today. Operators ensure that water quality standards are always being followed and that operations throughout the facility, like the aeration tanks and incineration, are functioning properly. Lake Ontario is our most important body of water. It is the home to a variety of fish and plant life. It's the source of our drinking water, and, as we saw today, where stormwater and treated wastewater ends up. Even though our wastewater goes through an intense treatment process, we want to limit our impact on the environment. We can do this by keeping harmful chemicals, like paints, expired medication, and harsh cleaners, out of our sinks, drains, and toilets. Let's all do our part to keep Lake Ontario clean and healthy.
As we can see from these videos, the design of a large-capacity water treatment plant is very complex and involves not one but many steps, each of those utilizing multiple technologies. It is not our goal to learn all of them in detail in one lesson. However, should you have a specific interest in this topic, the US EPA Wastewater Technology Fact Sheets web page can serve as a great resource for obtaining more technical information about them.
Depending on the degree of cleaning and purification, treated water can be reused for:
- irrigation of agricultural crops or landscape irrigation (e.g., schoolyards, golf courses, residential gardens);
- groundwater recharge;
- recreational use (e.g., lakes and ponds, fisheries, snowmaking, marsh enhancement);
- non-potable urban use (e.g., fire protection, air conditioning, toilet flushing);
- potable use;
- industrial use (e.g., cooling, process water).
The main concern in water reuse is to meet the water quality requirements for its intended use. Quality requirements are determined by federal, state, and regional regulatory authorities and may vary. The general guidelines by EPA with regards to the effluent from the wastewater treatment facilities are given in Table 6.2 below:
Measures | Effluent Limitation | ||
---|---|---|---|
Daily maximum | Weekly average | Monthly average | |
5-day Biochemical Oxygen Demand (BOD5) | 60 mg/L | 45 mg/L | 30 mg/L |
5-day Carbonaceous Biochemical Oxygen Demand (CBOD5) | 50 mg/L | 40 mg/L | 25 mg/L |
Total Suspended Solids (TSS) | 60 mg/L | 45 mg/L | 30 mg/L |
pH (instantaneous) | 6.0-9.0 | --- | --- |
Here is an explanation of measures in this table if you are not familiar with the terms:
- BOD5 is the amount of oxygen needed to oxidize organic matter in a water sample. The difference in oxygen content is usually measured over the time span of 5 days (that is the reason for subscript 5). For reference, water from a very clear source may have a BOD of less than 2 mg/L; sewage water may give readings above 100 mg/L; food processing wastes may have BOD of thousands.
- CBOD5 is the amount of oxygen needed to oxidize carbonaceous organic matter (excluding nitrogen compounds)
- TSS is the amount of particulate matter (insoluble) present in a water sample, which is usually determined by filtering the solution and weighing the residue remaining on the filter.
- pH is the measure of acidity of solution in chemistry (defined as pH = -log[H+]). Acidic solutions, such as acid rain, may have pH around 1-2, relatively neutral solutions range within 5-9 (distilled water pH=7), and alkaline solutions have pH 10-14.
These limits determined by EPA are included in the government regulations, published in the Rules of Department of Natural Resources [CSR, 2014]. This document also contains extensive data on limitations imposed on the contents of the toxic element in water before it is reused or discharged in a certain way to the environment. Check Table A for the maximum tolerated concentrations of metals (p.24) and organics (p. 26). The toxicity requirements are especially relevant to industrial water use.
Chemical tests to determine the above metrics are used as controls at any wastewater treatment plant. Various technologies are developed to improve the treatment efficiency and to produce a cleaner effluent suitable for further use.
Engineered ecological systems for water treatment
Traditional water treatment plants accomplish an important function. However, these facilities themselves produce significant environmental impact by consuming energy, producing emissions, by-products, and waste to be disposed of. Later in this lesson, an example is given for a life cycle assessment study which analyzes the way to make these systems more benign.
One of the trends in improving the environmental profile of wastewater treatment facilities is the design of ecological systems that mimic natural processes of neutralizing the pollution.
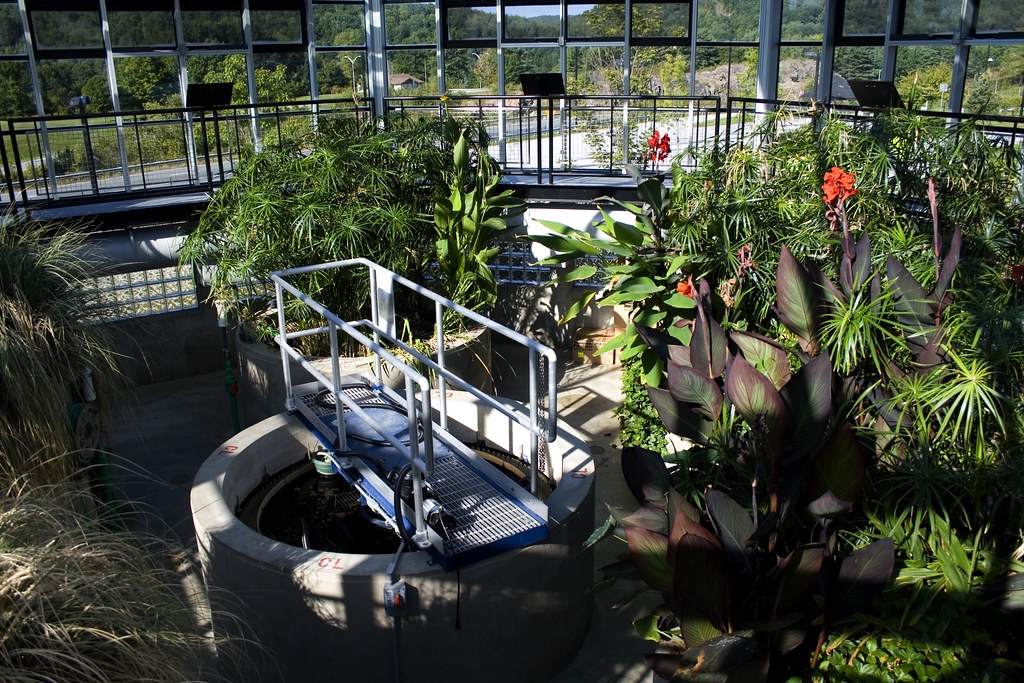
Here are a couple of examples of the development of such systems:
- Living Machine and Biomatrix systems (see photo above):
- Eco-Machines systems - ecological fluidized bed, or a small constructed wetland
These examples show that ecological treatment systems typically work at the small scale being capable to treat liquid waste from a community of 300-1000 people. This makes them attractive for decentralized treatment for secluded autonomous areas.
Decentralized systems for water treatment
Decentralized systems of water purification often become technologies of choice in developing countries because they do not require huge infrastructure or can be set up quicker when infrastructure is destroyed. Small-scale technologies provide quick response to urgent needs. There are multiple ways to approach the issue. Here is one of them: watch this 10 min video to see an example how small-scale technology can help solve large-scale problems.
Video: How to make filthy water drinkable (9:13)
Good morning, everybody. I'd like to talk about a couple of things today. The first thing is water. Now I see you've all been enjoying the water that's been provided for you here at the conference, over the past couple of days. And I'm sure you all feel that it's from a safe source.
But what if it wasn't? What if it was from a source like this? Then, statistics would actually say that half of you would now be suffering with diarrhea. I talked a lot in the past about statistics and the provision of safe drinking water for all. But they just don't seem to get through. And I think I've worked out why. It's because, using current thinking, the scale of the problem just seems too huge to contemplate solving. So, we just switch off: us, governments and aid agencies. Well, today, I'd like to show you that through thinking differently, the problem has been solved. By the way, since I've been speaking, another 13,000 people around the world are suffering now with diarrhea. And four children have just died.
I invented Lifesaver bottle because I got angry. I, like most of you, was sitting down, the day after Christmas in 2004, when I was watching the devastating news of the Asian tsunami as it rolled in, playing out on TV. The days and weeks that followed, people fleeing to the hills, being forced to drink contaminated water or face death. That really stuck with me. Then, a few months later, Hurricane Katrina slammed into the side of America. "Okay," I thought, "here's a First World country, let's see what they can do. "Day one: nothing. Day two: nothing. Do you know it took five days to get water to the Superdome? People were shooting each other on the streets for TV sets and water. That's when I decided I had to do something.
Now, I spent a lot of time in my garage, over the next weeks and months, and also in my kitchen -- much to the dismay of my wife. (Laughter) However, after a few failed prototypes, I finally came up with this, the Lifesaver bottle.
Okay, now for the science bit. Before Lifesaver, the best hand filters were only capable of filtering down to about 200 nanometers. The smallest bacteria is about 200 nanometers. So, a 200-nanometer bacteria is going to get through a 200-nanometer hole. The smallest virus, on the other hand, is about 25 nanometers. So, that's definitely going to get through those 200 nanometer holes. Lifesaver pores are 15 nanometers. So, nothing is getting through.
Okay, I'm going to give you a bit of a demonstration. Would you like to see that? I spent all the time setting this up, so I guess I should. We're in the fine city of Oxford. So -- someone's done that up. Fine city of Oxford, so what I've done is I've gone and got some water from the River Cherwell, and the River Thames, that flow through here. And this is the water. But I got to thinking, you know, if we were in the middle of a flood zone in Bangladesh, the water wouldn't look like this. So, I've gone and got some stuff to add into it. And this is from my pond.
(Sniffs) (Coughs) Have a smell of that, mister cameraman.
Okay. (Laughs) Right. We're just going to pour that in there.
Audience: Ugh!
Michael Pritchard: Okay. We've got some runoff from a sewage plant farm. So, I'm just going to put that in there. (Laughter) Put that in there. There we go. (Laughter) And some other bits and pieces, chuck that in there. And I've got a gift here from a friend of mine's rabbit. So we're just going to put that in there as well. (Laughter) Okay. (Laughter) Now.
The Lifesaver bottle works really simply. You just scoop the water up. Today, I'm going to use a jug just to show you all. Let's get a bit of that poo in there. That's not dirty enough. Let's just stir that up a little bit. Okay, so I'm going to take this really filthy water, and put it in here. Do you want a drink yet? (Laughter) Okay. There we go. Replace the top. Give it a few pumps. Okay? That's all that's necessary. Now, as soon as I pop the teat, sterile drinking water is going to come out. I've got to be quick. Okay, ready? There we go. Mind the electrics. That is safe, sterile drinking water. (Applause) Cheers. (Applause) There you go, Chris. (Applause)What's it taste of?
Chris Anderson: Delicious.
Michael Pritchard: Okay. Let's see Chris's program throughout the rest of the show. Okay? (Laughter)
Okay. Lifesaver bottle is used by thousands of people around the world. It'll last for 6,000 liters. And when it's expired, using failsafe technology, the system will shut off, protecting the user. Pop the cartridge out. Pop a new one in. It's good for another 6,000 liters.
So, let's look at the applications. Traditionally, in a crisis, what do we do? We ship water. Then, after a few weeks, we set up camps. And people are forced to come into the camps to get their safe drinking water. What happens when 20,000 people congregate in a camp? Diseases spread. More resources are required. The problem just becomes self-perpetuating. But by thinking differently, and shipping these, people can stay put. They can make their own sterile drinking water, and start to get on with rebuilding their homes and their lives.
Now, it doesn't require a natural disaster for this to work. Using the old thinking, of national infrastructure and pipework, is too expensive. When you run the numbers on a calculator, you run out of noughts. So, here is the "thinking different" bit.
Instead of shipping water, and using man-made processes to do it, let's use Mother Nature. She's got a fantastic system. She picks the water up from there, desalinates it, for free, transports it over there, and dumps it onto the mountains, rivers, and streams. And where do people live? Near water. All we've got to do is make it sterile. How do we do that?
Well, we could use the Lifesaver bottle. Or we could use one of these. The same technology, in a jerry can. This will process 25,000 liters of water; that's good enough for a family of four, for three years. And how much does it cost? About half a cent a day to run. Thank you.
So, by thinking differently, and processing water at the point of use, mothers and children no longer have to walk four hours a day to collect their water. They can get it from a source nearby. So, with just eight billion dollars, we can hit the millennium goal's target of halving the number of people without access to safe drinking water. To put that into context, The U.K. government spends about 12 billion pounds a year on foreign aid. But why stop there? With 20 billion dollars, everyone can have access to safe drinking water. So, the three-and-a-half billion people that suffer every year as a result, and the two million kids that die every year, will live. Thank you.
Click on the link below to read about some small innovations that make big difference when applied at the right place at the right time:
6 Water-purifying Devices for Clean Drinking Water in the Developing World
Reading Assignment:
Next, let us get a little bit deeper into the issue. I ask you to read the following paper which analyzes a case study of a decentralized wastewater treatment system in India. It covers enough technical details to understand how the technology works and provides a useful discussion of environmental, economic, and social aspects:
Dhinadhayalan, M., Nema, A., Decentralised wastewater management - New concepts and innovative technological feasibility for developing countries, Sustain. Environ. Res., 22(1), 39-44 (2012). (The full article is accessible via Library e-Reserves in Canvas)
While reading, focus on understanding the pros and cons of the decentralized approach (compared to central distribution system). In your own notes, list three key advantages of decentralized technologies that justify their development and implementation. Also, list three disadvantages that may limit their use in different parts of the world. Can you imagine to use only de-centralized water treatment in your current location? What kind of social impacts might it create?
Check Your Understanding
The BOD is a measure of which type of pollutant in the water?
Click for answer.
Organic matter; BOD is the amount of oxygen needed to oxidize the organic compound in a given volume of water.
Check Your Understanding
Which stages in wastewater treatment are designed to decrease BOD of the effluent?
Click for answer.
Aerobic biological (bacterial) decomposition. Bacteria in a well-aerated pool are capable of decreasing BOD by over 90%