
2.1 Technology as a part of anthropogenic environment
How do we define technology? In this course, specifically, we need to look at a particular technology, process, or product as an active part (component) of an anthropogenic system. In such context, a technology is not simply a piece of human knowledge implemented through design and engineering principles, it is considered a "living" part of a bigger organism. Here, we use the word "living" because our interest will be to assess the entire lifecycle of a technology: development, adaptation, operation, interactions with natural and technical environment, aging, and death (in some cases). Further on, we will try to understand how technology development impacts the viability of the whole system.
The common definition of the term technology is quite broad and multi-colored. The most simplistic one is application of scientific knowledge for practical purpose. And as an extension of it, the tool or device enabling that application is typically also referred to as technology. You can read more on the history and usage of this term in a Wikipedia article on Technology. You may recognize that the meaning strongly depends on the context and the professional area you are in. However, in this course, we need to distill this broad perception of technology to a more specific entity that can be used for practical analysis.
Energy and matter conversion
The most important ability of almost any technology is conversion. A technology uses inputs of energy or matter to create outputs of energy or matter of a different quality. In a general case, any technology can be represented by the following scheme (Figure 2.1):
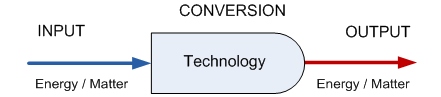
So, technology typically serves as a conversion portal in a system. We use energy to produce materials; or use raw materials to produce some more complex products; or we use matter to convert forms energy; etc. Note that conversion can also be performed by natural systems or mechanisms; but we only define technology as a human-made conversion system.
Here are some simple examples:
- Chemical energy of fuel >>> Car >>> Kinetic energy of car motion
- Radiative energy (sunlight) >>> Solar panel >>> Electric energy
- Electric energy >>> Phone >>> Sound, light
- Contaminated water >>> Water treatment plant >>> Clean water
- Electric energy >>> Furnace >>> Thermal energy
- Flour, water >>> Baking machine >>> Bread
- Organic waste >>> Composting >>> Fertilizer
- Electricity, mechanical energy >>> Electric guitar >>> sound
You can continue this list.
Self-Check
See if you can identify the energy and matter inputs and outputs for the following technologies:
- ? >>> Coffee maker >>> ?
- ? >>> Battery >>>> ?
- ? >>> Loud speaker >>> ?
- ? >>> Microwave >>> ?
ANSWER
- Water, ground coffee, electricity >>> Coffee maker >>> Coffee Drink
- Chemical energy >>> Battery >>>> Electricity (upon discharge)
- Electricity >>> Loud speaker >>> Sound
- Electricity >>> Microwave >>> Microwave radiation, kinetic energy (rotation)
Conversion efficiency
Obviously some technologies are better converters than others, and the following metric allows us to compare different technological options and choose a "better deal" in terms of useful output and money spent.
The key characteristic of any conversion process is efficiency. Efficiency is estimated based on the amount of useful output per unit input. In that sense, it is a subjective value which depends on a particular goal or purpose of a technological process, and a particular input resource we are concerned about. Hence, efficiency has widely varying meanings in different disciplines.
For example, efficiency is a very common metric in the field of energy conversion. According to the energy conservation law, the total energy entering a conversion device should be equal to the total energy output by the device:
Ein = Eout
Some of the output energy can be considered useful (based on the purpose of conversion), and some of it can be considered not useful and attributed to "losses":
Eout = Eout(useful) + Eout(loss)
What is useful and what is not is up to us to define (nature does not care!).
So, efficiency determines the fraction of the useful energy as follows:
Efficiency = Eout(useful) / Ein × 100%
Efficiency is important in the sustainability context because it indicates how much of the resource is put to work, and how much of the resource is wasted in the process. The reasons for losses are process dependent and should be analyzed specifically for each application. A big part of the technological research is aimed at increasing efficiency of the conversion process via minimizing losses.
Example 1
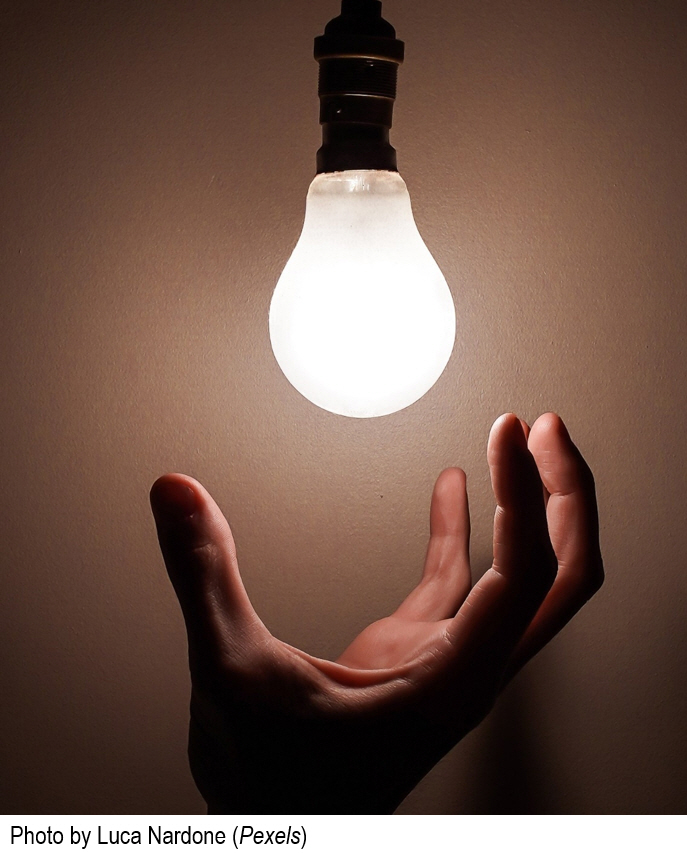
A typical incandescent light bulb outputs both light and heat. If you ever touched the working light bulb with a bare hand, you know that there is a good amount of heat generated in this kind of energy conversion.
If I use the bulb to lit my dining area, the useful energy I collect is obviously light, or radiant energy, and efficiency of the conversion process would be defined as:
Efficiency = (Light Output / Electricity Input) x 100%
But if I use the bulb to warm my home incubator (with eggs waiting to hatch), the useful energy in this case would be heat. And the generated light would be in fact unnecessary, that is not useful output. In this case the efficiency of the conversion process can be defined as:
Efficiency = (Heat Output / Electricity Input) x 100%
Here we can see that efficiency is often defined in the eye of the beholder.
By the way, the efficiency of the incandescent bulb in the first case is much lower than in the second. Conversion to light is on the average 2.2% efficient, while the rest of input energy (97.8%) goes into heat.
Example 2
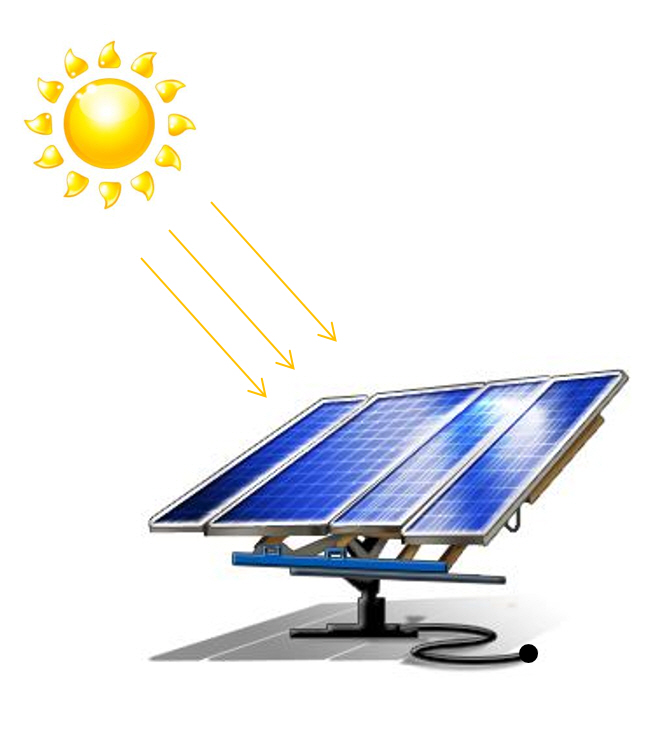
As another illustration of how this concept works, let us estimate the efficiency of a photovoltaic panel. Photovoltaic technology converts visible solar radiation (energy-in) into electric power (energy-out). So, for this estimation, we need to know or measure these two quantities.
Let us assume that the efficiency is measured in the middle of a sunny day, and the panel is installed perpendicular to the incident rays. Under those conditions, the typical incident radiation flux is ~1000 W/m2. We can take this number as the measure of energy-in per unit of time.
Now, let us assume that the panel outputs the power density 120 W/m2. Usually, this value can be obtained by measuring the voltage and current density of the panel (power = voltage x current).
Then, the efficiency value can be calculated as follows:
Efficiency = Eout(useful)/Ein × 100% = 120 W/m2 / 1000 W/m2 × 100% = 12%
This value means that 88% of total solar energy reaching the panel is lost, and only 12% is converted to electricity due to technology limitations or environmental factors. Just FYI, the nominal efficiency of most solar panels on market ranges between 15 and 22% (under ideal conditions). As you can see, efficiency estimations require data on technical performance of the system, so we will be paying attention to how performance of different technologies can be measured and interpreted.
Table 2.1 lists some known efficiencies of various energy technologies for comparison. These are just a few examples to demonstrate the variety of converters. We should note that generally efficiency of a process or technology is not necessarily measured in terms of energy. If the useful output of the converting technology is, for example, some form of matter (e.g., water electrolyzer in this table), the calculation can be made in terms of mass. Sometimes, efficiency analysis is also used to estimate the maximum theoretical efficiency, which cannot be practically exceeded due to inherent physicochemical limitation of the system. Finding maximum theoretical efficiency requires detailed knowledge of how the process works and what unavoidable losses occur in conversion.
Process or Technology | Input | Useful Output | Conversion efficiency |
---|---|---|---|
Gas turbine | Gas flow | Electricity | 40% |
Water turbine | Water flow | Electricity | 90% |
Solar cell | Light | Electricity | 15-40% |
Fuel cell | H2(gas), O2(gas) | Electricity | up to 85% |
Water electrolyzer | Electricity | H2(gas), O2(gas) | 50-70% |
Combustion engine | Fuel (gasoline) | Motion (kinetic energy) | 10-50% |
Geothermal electric plant | Heat | Electricity | 10-23% |
Solar thermoelectric generator | Sun radiation | Electricity | 15% |
Electric motor | Electricity | Motion (kenetic energy) | 30-90% |
Electric heater | Electricity | Heat | up to 100% |
Refrigerator | Electricity | Negative heat | 20-40% |
Fluorescent lamp | Electricity | Light | 8-15% |
Photosynthesis | Light | Biomass, O2(gas) | 3-6% |
Muscle | Metabolic energy | Kinetic energy | 18-25% |
Probing Question
Can energy conversion efficiency be more than 100%? Click on answer below.
YES
NO
Yes, but only in an ideal zero-loss system
Probing Question
Can you calculate the efficiency of an electric motor that consumes 150 W of electrical power and produces 120 W of mechanical power? Click on answer below.
8%
65%
80%
125%
Technology Adaptation
To become part of society life, technology needs to be adapted. Not all technologies invented go through successful adaptation, and there are several critical barriers that need to be overcome in order to create a working interface between technology and society.
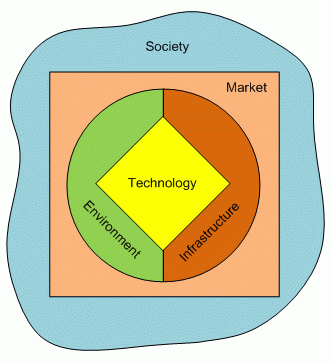
Consider the following stages of technology adaptation:
- Technical Adaptation. Research and development, design, and demonstration. (yellow)
- Adaptation to the natural environment: matter and energy exchange, use of resources, and absorption of the impact (green).
- Adaptation to the technical environment: development of infrastructure and supporting technologies (brown).
- Market Adaptation. Development of economic algorithm. Proof of profit and long-term feasibility (peach).
- Social Adaptation. Acceptance by target society groups, based on ethical, environmental, cultural, and economic considerations (blue).
The first three stages of adaptation can be reflected in more detail through the Technology Readiness Level scale (TRL) on page 2.2 of this lesson. The fourth stage of adaptation is closely related to economic assessment, which should answer the question if the technology can support itself and make a profit in the short term or in the long term. Finally, the fifth stage of adaptation includes multiple social factors - how ready consumers are to accept this new technology, and what socal benefits it promises (for example, higher standard of living, job creation, convenience, faster service, improved health, etc.). The sustainability analysis should be comprehensive enough to cover all of these layers of adaptation and recognize connections and feedbacks between them.