
Sedimentary Rocks
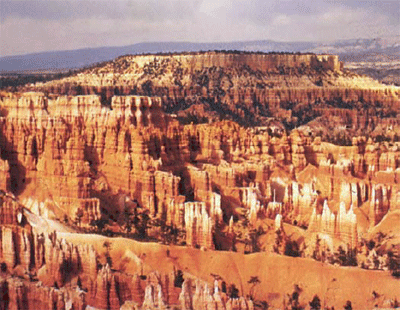
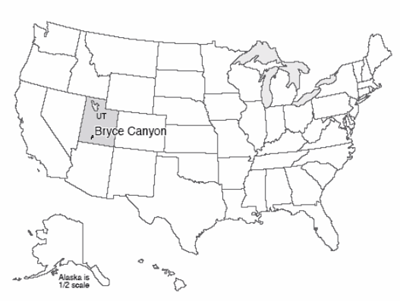
Bryce Canyon is a fairyland. Pillars, spires, and minarets, seemingly fragile and toy-like when viewed from the rim, tower far above the hiker who descends into the “canyon,” which is really a bowl carved out of the edge of a plateau. The morning sun, bouncing off the pink and pastel walls, casts a rosy glow on everything and everyone in the canyon. Gnarled trees cling to high slopes, their root structure exposed by erosion but somehow holding and nourishing the twisted branches. The old settler Ebenezer Bryce called the scenery of this tributary of the Paria River “a hell of a place to lose a cow,” but almost everyone who followed him has had a kinder assessment.
The main pink rocks of Bryce are a mixture of limestones, shales, and some coarser sandstones and conglomerates, deposited in a lake or a series of lakes, and together known as the Claron Formation or the Bryce Limestone. (Geologists studying regions have found it useful to give specific names to recognizable bodies of rock, with the names taken from geographic locations. Because the criterion for a new name is the ability to easily recognize a unit in the field, some really careful observers like to subdivide, and others like to lump, so there is often slight disagreement as to which name is most appropriate.) These Bryce rocks are from the early part of the Cenozoic, roughly 40 million years ago. The rocks record a generally wet time shortly following uplift of the land from a shallow seaway, and before continued uplift led to the high plateaus of today, and climate change dried the surroundings to the dry climate now at Bryce.
Some of this story is told in the rocks of Bryce. The rest is told nearby. Northeast of Bryce in central Utah, the rocks record the gradual transition from undersea to terrestrial. The growth and erosion of mountains to the west are marked by the appearance and then disappearance of coarse braided-stream deposits. These were replaced by slower streams, then by lakes, then another pulse of river sediments, and then the great Green River lakes that spread from Bryce to Wyoming and that record a wet, warm time in the west.
Geologists love to tell stories like this one. The seas came in, the seas went out, the mountains rose, the mountains were eroded. Such stories are useful, because they often include chapters such as “The oil formed here, and migrated there, and was trapped in those rocks, and we can drill into it this way and get rich.” Such stories tell us much about the stability of the Earth’s climate. And the stories are interesting to many people. But how do you read such stories, and gain confidence in them? One way to find out is to major in Geosciences and spend the next three years learning to do so professionally—in case you’re interested, talk to us! But if you’re really excited about the great career prospects in your current major of theatre or philosophy, at least read over the next few sections and you’ll get a brief sketch of geological techniques and what they tell us.
We saw at the Badlands that weather attacks and breaks down rocks, and we saw at the Tetons, in Canyonlands, and elsewhere that the loose pieces are transported. The processes of weathering and erosion produce new minerals from old, produce small pieces from big ones, produce dissolved salts, and move these around on the surface of the Earth. Eventually, these materials end up somewhere. The pieces derived from older rocks we call sediment. Sediment may be deposited on the bottom of a sea, or on the flood plain of a river, or at the end of a glacier. Sediment may stay for a while and then be moved on. If it stays for too long, it may be hardened.
There is no magic in making rock from sediment, nor is there an abrupt line separating the two. Many processes slowly transform loose pieces into pieces stuck together. If you don’t believe that loose pieces get stuck together, go to an old house and try to remove some piece of plumbing without using a really big wrench, or try to get pieces off an old bicycle without a big wrench. If you don’t have the time, trust us—people make big wrenches for a good reason! Nature does rust and otherwise bond things together in many cases.
The weight of additional material deposited on top of a sediment layer will squeeze it, forcing grains together and pushing water out. The resulting material is harder than what was there before. Continued squeezing and heating as sediment is buried (remember that the Mississippi delta is miles thick) produce rock from this sediment, and eventually may change these sedimentary rocks to metamorphic rocks. The clays in sediment change into different clays and then into other things as the cooking proceeds (just as flour and water and yeast make cookies in the oven), and as the crystals of the new minerals grow, they tend to wrap around each other and get “woven” together so they are hard to separate.
Groundwater circulating through sediments will dissolve minerals in some places and precipitate them in others, in response to subtle changes in temperature, pressure, or chemistry. We saw that there is dissolved rock in Spring Creek water, and in all other waters. If you have “hard” water, of the sort that causes people to buy water softeners, that means the water has a lot of dissolved rock and especially dissolved limestone. The white deposit that builds up on drinking glasses in houses with hard water is not soap scum but rock, very similar to stalactites in a cave. A lot of the sticking-together of plumbing pieces is linked to minerals precipitated from hard water. What happens in your house can happen in nature, where the precipitate serves as a cement that binds particles together. This cementation, together with the compaction from squeezing and the recrystallization from heating and changing clays, slowly turn loose sediment to sedimentary rock. Sometimes, rocks are made entirely of hard-water deposits—stalactites are an example, but so are the salt deposits left in the bottom of Death Valley when the water that runs down from the peaks quickly evaporates. Rocks made entirely of precipitate are also called sedimentary rocks.
Classification of Sedimentary Rocks
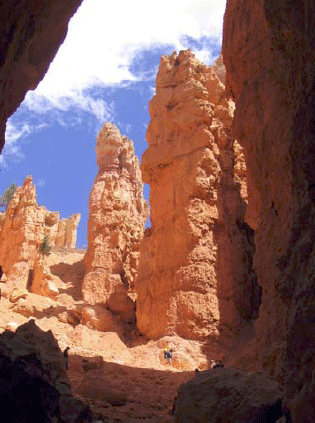
Our classification of sedimentary rocks is a bit of a hodge-podge. We first consider whether a rock is clastic (made from pieces or clasts of older rocks) or chemically precipitated (deposited from chemicals dissolved in water). This subdivision is not always satisfactory—a sea shell is a chemical precipitate because the animal pulled the material in its shell from the water, but a limestone made up of sea shells might be called clastic because the sea shells are chunks. Usually, people consider limestones and evaporites (rocks left by evaporation of water containing salts) to be chemical precipitates, and all others to be clastics.
Clastics are classified based primarily on grain size. The very smallest particles of clay make claystone or shale. Slightly coarser pieces are silt and make siltstone. Coarser still is sand, which makes sandstone. Going to still-bigger clasts, cobbles and boulders produce cobblestone and boulder-stone, but we also call both of these conglomerate.
Reading Sedimentary Rocks
We learn about the past through sediment. Archaeologists dig up the garbage dumps and houses of past peoples to learn how they lived. Historians root through the intellectual sediment of people to learn what they have done. Sedimentary rocks, in the same way, tell the history of the surface of the Earth.
A typical sedimentary rock contains all sorts of clues as to how it came to be. The clast size or grain size is a measure of the energy of transport—tiny clay particles will not settle out of a swift mountain stream, so a fine-grained deposit indicates slow or no currents. Grain shape tells more—an angular clast has not been transported far in water or wind, because during transport sharp corners are knocked off and the clast becomes rounded. Sorting also tells something—wind and water sort sediments by size, but landslides and glacier ice do not, so the sand grains on a beach are all about the same size, but a landslide leaves a deposit that ranges from miniscule clay-sized particles up to house-sized boulders and houses.
Composition provides clues—olivine is a mineral that is changed rapidly by chemical weathering and occurs primarily in low-silica igneous rocks such as sea-floor basalts, so if you find olivine grains in a sandstone, you know both that the sand was derived from such rocks, and that the sand was not transported very far in a wet environment because the olivine was not broken down. Even surface textures have information—the striations and polish of a glacier-transported rock look very different from the sand-blasted appearance of a quartz grain in a sand dune.
The sizes and shapes of deposits give further information. There are several ways to tell which way a current of wind or water was moving when it deposited a particular rock. For example, with sand dunes, the wind carries sand up a gradual slope and then dumps the sand over the top where it avalanches down and forms a steeper slope. When we find the gentle and the steeper slope in the rock, we can tell which way the wind was blowing. Sand ripples and bars deposited by flowing water have similar indicators. A sand body deposited by a stream often is long and skinny in the direction that the stream was flowing. A “fossil” sandy beach may be long and skinny like a stream deposit, but the current directions caused by waves will be pointing more-or-less across a beach but along a stream, so there is not much danger of confusing one type of deposit for another.
The list of indicators is very long. When mud dries out, it cracks to form mud cracks. Such features are easily recognized in rocks, and indicate occasional drying. Raindrops that spatter down on rocks leave little pock marks that are readily recognized if they are turned to stone and preserved.
Added to this, we have the advantage of fossils. Tree trunks in growth position indicate a non-desert deposit on land—trees are replaced by cactus in desert environments, and by seaweed underwater. Coral skeletons indicate a reef in the ocean.
With such a wealth of information, we certainly should be able to read much of the story of the past. All that is required is that: 1) we study the rocks carefully; 2) we know what sorts of sediments are produced in what sorts of environments today; and 3) we make the common-sense assumption of “uniformitarianism”, that the present is the key to the past.
Suppose that you go out today and observe that streams flow downhill and carry rock and mud from the hills to the sea. You dig around in a lot of different streams, and find that they have sorted sedimentary beds, occasional fish fossils, current-direction indicators pointing more-or-less the same way in many different sand bars, sand bars of certain shapes and sizes, etc. You talk to a lot of people, and read history books, and learn that streams have been flowing downhill and carrying rock and mud from the hills to the sea for a long time. You find a place where earlier people had diverted a stream into a canal, dig up the old stream bed, and find that it looks just like modern stream beds. You find still older deposits that look exactly like modern stream beds, and you find deposits in which the sand has been cemented by hard-water deposits to make sandstone, but everything else looks just like modern stream beds.
The reasonable person would say that these “fossil” cemented-to-stone stream beds are indeed old stream beds. Of course, we cannot prove that they are. Perhaps space aliens made the world the year before you were born in such a way that it looks like there were old streams, and the aliens invented all the history books and fake memories of old people. Calvin (of the Calvin and Hobbes comic strip) once told Susie that their class was having a substitute teacher because “our REAL teacher rocketed back to Saturn to report to her superiors. They’re trying to subvert us little kids with subliminal messages in our textbooks, telling us to turn in our parents when the Saturnians attack. Earth will be rendered helpless! I’m too smart for ‘em, though! I don’t read my assignments!” (To which Susie replied, “I think one of us has been eating too much paste in art class.”) Maybe Calvin is right. And maybe the Geology-of-National-Parks professors are Saturnian aliens, too! The textbook author, Dr. Alley, has really strange-looking hair where it hasn’t fallen out, and acts a bit peculiarly, you know.
However, despite his occasional interest in the topic, Calvin had not quite mastered geologic thought. Geologists take the common-sense approach: if it looks like a duck, and walks like a duck, and quacks like a duck, is genetically identical to type specimens of ducks, has a bone structure as observed in x-ray examination that matches known duck bone structure, mates with other ducks and produces duck offspring, etc., we call it a duck. If it looks like a stream deposit in size, shape, arrangement, location, grain size, sedimentary structures, fossils, etc., we call it a stream deposit. We learn from the present—what do streams do, what do they make, where do they occur—and then we use that knowledge as the key to interpreting the past.
Learning from the present is not a casual undertaking. By working hard in one or two advanced courses, a really adept student can learn to distinguish most of the main deposits—streams versus lakes versus beaches, for example. Many geologists spend their whole lives really learning what stream deposits look like, how they form, etc., while other people are spending their lives working on beaches, or lakes, or glaciers.
When lots of these experts agree that the limestones of Bryce and surroundings were deposited in a shallow lake and adjacent streams and mud flats, you should know that the rock type (limestone, but with differences in chemistry and texture from limestones forming in oceans), the fossils (algae, fish fossils, alligator bones, bird tracks), the structures (mud cracks, raindrop imprints), etc., have been considered carefully in telling the story. We can’t rule out Calvin’s Saturnians, but a more down-to-earth explanation is more convincing.