
Additional reading from www.astronomynotes.com
Star clusters provide us with a lot of information that is relevant to the study of stars in general. The main reason is that we assume that all stars in a cluster formed almost simultaneously from the same cloud of interstellar gas, which means that the stars in the cluster should be very homogeneous in their properties. This means that the only significant difference between stars in a cluster is their mass, but if we measure the properties of one star (age, distance, composition, etc.), we can assume that the properties of the rest of the stars in the cluster will be very similar.
In reality, some stars in the cluster form earlier than others, but compared to their lifetimes, the spread in their formation times is small and can be ignored. We also assume that the stars in a cluster are all the same distance away from us. Again, there is in fact a spread in distance, but, in most cases, this spread is much smaller than the distance to the cluster, so it can be ignored. For example, the outermost stars in the globular cluster M13 are about 50 parsecs from the center of the cluster, but the cluster is about 7,700 parsecs away from us. Finally, we assume that the chemical composition of all of the stars in a particular cluster should be very similar because the cloud of gas from which they formed is expected to have been well mixed, so the individual cloud fragments that formed individual stars should all have contained the same mix of elements and molecules.
When stars form out of a molecular cloud, very high mass stars (perhaps up to about 100 times the mass of the Sun) all the way down to low mass, brown dwarf objects (about 0.08 solar masses) are formed. Observations of newly formed populations of stars have shown us that very few high mass stars form, while many low mass stars form. The drop-off is very steep as you get to higher masses, as well. If you were to survey the stars near the Sun, you would find that about 90% of all stars in our Solar Neighborhood are less than or equal to the Sun's mass. Most of the rest are less than twice the mass of the Sun, and only about 0.5% of all nearby stars are more massive than 8 times the mass of the Sun. Remarkably, observations of star formation in many different locations in the universe seem to indicate that the relative ratios of stars of different masses that form is a universal law. That is, the same relative proportion of high mass compared to low mass stars always forms regardless of the size of the star forming region, the environment in which the star forming region resides, and how long ago the stars formed. Therefore, if we can determine how one cluster of stars formed, we can generalize our findings to apply to all clusters. This idea of a relationship between the number of stars formed in a star forming region and their mass is referred to as the stellar initial mass function.
Let us follow the evolution of an entire cluster of stars through several stages of its lifetime.
This presentation (with credit to Penn State Astronomy & Astrophysics) allows you to click through slides that step you through the evolution. You will see a series of schematic HR diagrams for the stars in a cluster. In each frame, as the stars age, their luminosities and temperatures evolve, changing the overall appearance of the diagram with age.
The presentation progresses in the following way:
- At an early time stamp in the star cluster’s formation, what we will call t=0, most of the high mass stars have reached the Main Sequence, while some of the lower mass stars are still in the T Tauri phase.
- Ten million years (107 years) later, the highest mass O stars have used up all of their hydrogen and begin to evolve off the Main Sequence.
- After 100 million years (108 years), all of the O stars have gone supernova. The B stars begin to evolve off of the Main Sequence.
- After 1 billion years (109 years), All of the B stars that are massive enough have gone supernova and the rest have evolved into red giants. The A stars begin to evolve off of the Main Sequence.
- After 5 billion years (5x109 years), The G stars begin to evolve off of the Main Sequence. The red giant branch is populated with some of the originally more massive stars. Some of the first red giant stars that formed have already become white dwarfs.
- After 10 billion years (1010 years), The OBAFG stars are all missing from the Main Sequence, the red giant branch is very well populated, and there are also many white dwarfs. Only K & M stars remain on the Main Sequence.
What we see in the sequence is that as a cluster of stars ages, the top of the Main Sequence disappears first. The analogy you often hear is that it is like the wick of a candle—as the cluster stars burn out, the Main Sequence gets shorter. Therefore, if you can identify exactly what type of star is just now undergoing the transition from Main Sequence to red giant (called the Main Sequence Turn-Off), and if you know how long (theoretically) that it takes stars of that type to use up all of their hydrogen, you can estimate the age of that star. Now, because we assume that all of the stars in the cluster formed simultaneously, we can assume that all stars in the cluster have the same age as the most massive star left on the Main Sequence. Astronomers frequently use this technique of Main Sequence Turn-Off fitting to estimate the age of star clusters. The way this is done in practice is the following:
- Astronomers use computer models to create a theoretical HR Diagram for a population of stars with a specific age, say 500 million years. Instead of plotting the individual points, they plot a line that goes through the points of all of the stars in the HR diagram. Since this line indicates the positions of stars with a specific age, it is called an isochrone.
- Astronomers plot the observed colors and luminosities for the stars in a star cluster.
- You find the best match between a theoretical isochrone and the stars in your cluster, and that tells you the age of the cluster.
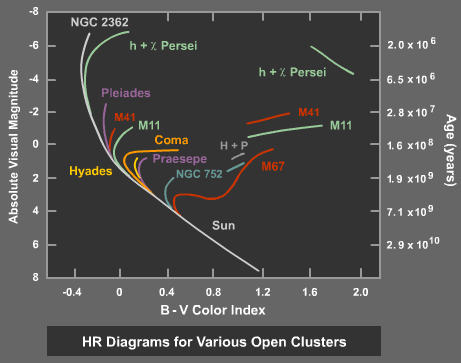
If you compare the HR diagrams for stages 1-3, these are very similar to the HR diagrams for open clusters. The HR diagram for stage 6 appears to be very similar to that of a globular cluster. Thus, we can conclude that open clusters are young (usually a few tens of millions or hundreds of millions of years old), while globular clusters are very old (typically about 12-13 billion years old). In the image above, you can see a schematic HR diagram with plots of lines that represent the Main Sequence for a number of open clusters. From the location of the Main Sequence Turn-Off, you can see that NGC 2362 is the youngest, then h & χ Persei, and M67 is the oldest of the clusters.
This realization explains several of the other observations that we made of the differences between these two types of clusters. Since open clusters are young, they have not had a chance to move very far from the location where they were born. Thus, there is likely to be leftover material from the molecular clouds in which they formed nearby (which creates the reflection nebulae seen in the Pleiades). The intense radiation from the bright O & B stars in the open clusters can ionize the nearby gas, creating emission nebulae nearby, as well. The light from an open cluster is dominated by the brightest stars in the cluster, which are O & B Main Sequence stars, since no red giants have formed yet. Thus, open clusters should be quite blue.
Since globular clusters are old, they are not found near the regions in which they formed. There is no gas in their vicinity. Even if there was, the stars in globular clusters do not emit much ultraviolet light capable of creating emission nebulae. Thus, we do not expect to find emission nebulae surrounding globular clusters. There are no blue Main Sequence stars left, so the light from a globular cluster should be dominated by the brightest red giants, leading to their very red appearance.
Lastly, we can also explain the difference in chemical composition between open cluster stars and globular cluster stars. Originally, all of the gas in the universe contained few elements heavier than helium. However, as we learned in our study of stellar evolution, heavier elements are created in massive stars and dispersed when they go supernova. Therefore, as time goes on, later generations of stars should contain higher and higher concentrations of heavy elements. Since globular clusters are 12 billion years old, their atmospheres reflect the makeup of the primordial gas from which they formed. Since open clusters have formed relatively recently, they have 10-100 times more heavy elements in their atmospheres.
One question that we have not yet answered is why the Sun is so apparently isolated. If all stars form out of molecular clouds that form many stars at once, why are there not several hundred stars nearby? The reason has something to do with the density differences between star clusters. Remember, open clusters look “fluffy. " That is, they are not very concentrated. Globular clusters, on the other hand, are very densely packed with stars. In open clusters, the gravitational pull of all the stars taken together is not strong enough to keep the stars bound to the cluster. Over time, the individual stars in open clusters drift away, and the cluster dissolves. The gravitational pull by the cluster on the stars in a globular cluster is much stronger, so these clusters are able to retain most of their stars for billions of years. It is likely that the Sun did form as part of an open cluster, but since the Sun is now about 5 billion years old, it has long ago drifted away from the stars that formed out of the same cloud.