Please watch the following video: 4:55
PRESENTER: In this module, we will be introducing the language of thermodynamics. Now, thermodynamics is a pretty wide subject with many different possible applications. So for the purpose of this class, we're just going to introduce a number of critical concepts on which a lot of our conversation will be founded.
And those critical concepts are the idea of energy, equilibrium, and the idea for system. Behind me is Kinzua Damn. And you can see in here that energy does not have to be a very abstract concept. Here you can see the water that is going to turn the turbines and produce electricity.
In other words, we're seeing energy in action. Pennsylvania gets a very large honor, in which it gets a whole geologic superior named after the State. And the reason for this is because of the abundance and the widespread presence of coal beds that form during this same period of time.
How do you make coal? Basically what happens is you take inland organic detritus, and you isolate it from the atmosphere and from the hydrosphere. At that point, you're going to cook it at a variety of temperatures and pressures. Depending on the temperature and depending on the pressure under which these materials form, you're going to get different grades of coal.
Now, this would be pretty ideal if you were to be forming only the coal. The complication is that just about every mineral forms as part of a rock. And when you have a rock, what you have is other minerals. Other minerals that you do not want if you're trying to extract coal for energy. So what this means is two things. It means that you have to separate those minerals from the coal, and it also means that you're going to be producing a large amount of tailings as a consequence of it.
Dangerous, in part, is that you can stop production of the coal. But those minerals that were present there are still going to be exposed to the conditions in the surface of the planet. And that can create a number of different problems.
We are outside of the city of Altoona in central Pennsylvania. And you may notice something unusual about this stream. Mainly, it is orange. This is colloquially known as yellow boy. And it forms by the deposition of iron oxides.
How do these iron oxides form? Well, this has to do with one of the major drivers of the local economy. And that has been the extraction of coal. As coal is extracted, we're moving from a closed system in the crust of the earth to an open system that is exposed to the atmosphere and exposed to groundwater and to surface waters.
So the consequences that that has is that the minerals that came along with the coal are going to react with the water and are going to react with the atmosphere. That will result in the breakdown or observation of many of the minerals that were present there. So one of the major culprits for the color of this material is the presence of an iron sulfide called pyrite.
Pyrite contains, basically, two atoms. Iron and sulfur. But when it oxidizes, it produces iron outside and it releases sulfur into the water. That results into acidification of the stream and death of the fish that were originally living in it. So you see that moving from one type of system to the other has consequences.
And also moving from a system that is in equilibrium under one set of conditions to another system in which you are completely out of equilibrium will result in pretty drastic consequences. So here we have two concepts of this chapter in thermodynamics being represented. The concept of open and close systems and the concept of equilibrium and this equilibrium.
"Everything should be made as simple as possible, but not simpler."
- Albert Einstein
To predict and measure the conditions under which sustainable production occurs, we analyze energy and material flows. A convenient way to describe these flows is using the language of Thermodynamics, which is the field of physics that studies the macroscopic aspects of energy and flows of energy and matter.
At the heart of Thermodynamics there are three concepts: Energy, Systems, and Equilibrium. We will be using those concepts throughout this course, and in particular in the next two lessons. So, this is a page to which you may want to return if some of that analysis becomes confusing. This is also a good reference because in order to define each of these three concepts, we need to introduce several other definitions.
Energy is the capacity to produce change. For computational purposes, we are normally concerned with the Internal Energy of system. There are many different forms of energy. Two of the most relevant forms of energy in engineering are Heat and Work. Heat is created by random motions of particles - a quantum mechanical idea, whose macroscopic outcome is differences in Temperature. Work is the capacity to move a mass over distance - back to classical mechanics!
You can create a running list of the forms of energy that you encounter in your everyday experience. That exercise will prove itself useful when we start working on energy and material flow analysis of systems, which brings us to the next concept.
The definition of a system is deceivingly simple: A System is the portion of the universe under study as defined by relevant energy and material flows (Fig. 3.1). Embedded in this definition is that the observer gets to choose the boundaries of the system under consideration. A system can be a computer chip, a factory, a country, or just about anything else.
A Phase is a restricted part of a system, with specific physical and chemical properties.
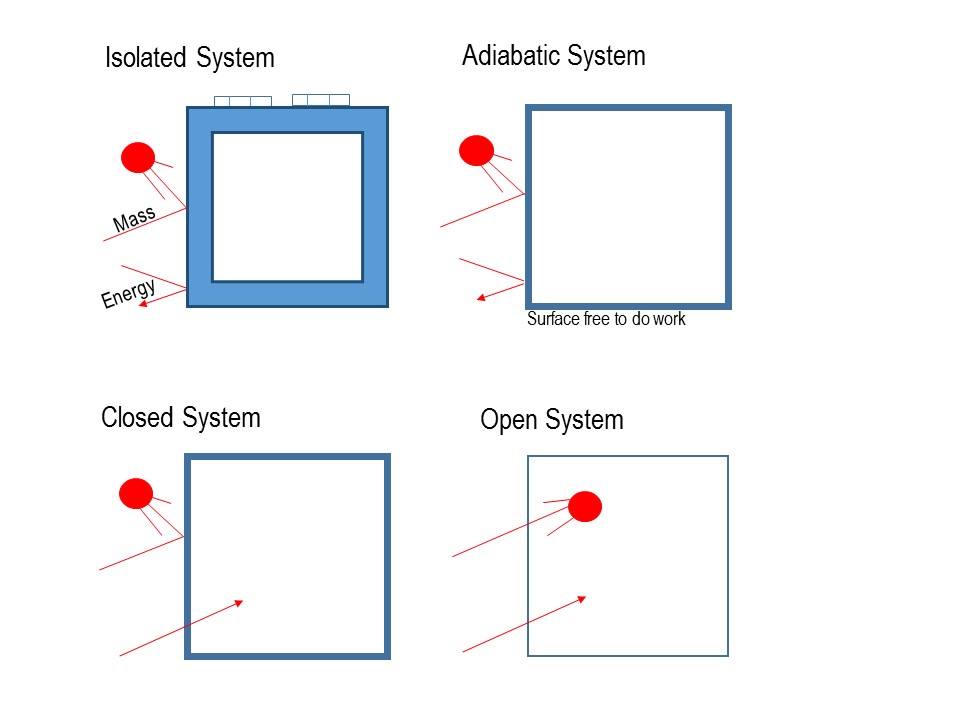
Here, the Surroundings are defined as everything else. What this means in practice is that in choosing systems with very narrow boundaries, you risk that the outcome of your analysis will take too long, be meaningless, or not fully and accurately predict the conditions under which environmental and social hazards can be prevented. Conversely, choosing too large of a system will make the system intractable.
In choosing a system, you are also tasked to choose how that system will interact with the surroundings. In Thermodynamics, you can choose from having (see Fig. 3.1):
- An Isolated System in which there is no exchange of any form of Energy or Matter. Clearly, this type of system does not exist in nature, but assuming an isolated system may be a helpful first order approximation to analyzing large or highly complex systems to a first approximation. In most cases, we are interested in how inputs to the system are transformed into outputs of a system. So, defining our system as isolated is not very helpful.
- An Adiabatic System does not have as severe limitations as an isolated system. In this type of system, there is no exchange of Heat and Matter. That is a system with thermally isolated boundaries - think the perfect refrigerator, the only problem being that you could never get your food out of it. In an adiabatic system, Work and other forms of energy can be exchanged with the surroundings.
- A Closed System is one in which all forms of energy are readily exchanged, but no material flows are allowed across system boundaries.
- Finally, in an Open System, both energy and matter can be exchanged with the surroundings.
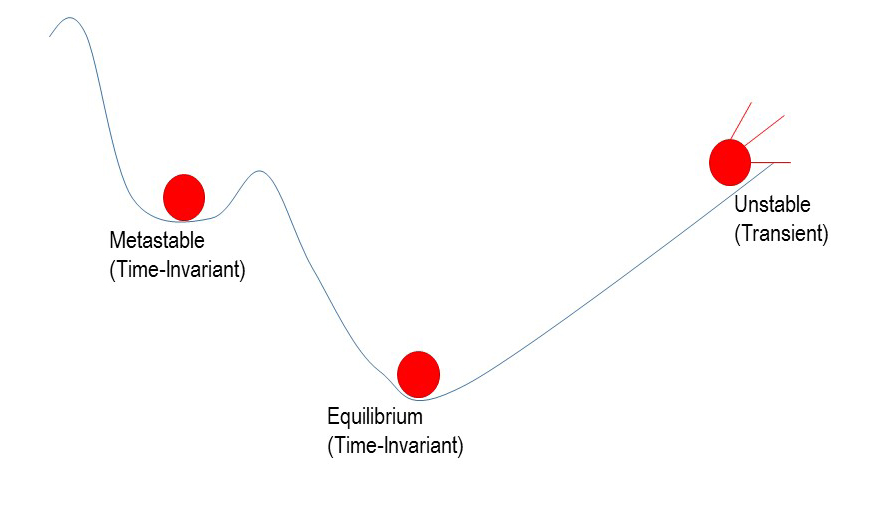
It is important to consider that a system may be closed for a particular variable and open for another. In minerals, for example, some chemical species are mobile while others are not. So the system would be considered open for the mobile species and closed otherwise. A system may also be considered closed for short time scales and open in long ones.
A system can either change with time or be time-invariant (Fig. 3.2). However, the Thermodynamics definitions are slightly different from our colloquial use of these terms. A system can be considered:
- Transient, if we are taking a snapshot of it. This definition allows us to evaluate when we can scale up our data and when it is not a good idea. For example, the concentration of air pollutants in a city can change drastically from rush hour to midnight. In this case, understanding that measurements are conducted in a transient system is critical to design a material flow analysis of the system.
- The other extreme is to consider that a system is Static and no change can occur.
- But, the matter and energy exchanges between the system and its surrounding cancel each other; the system is also time-invariant. This dynamic time-invariance is called Steady State. We will refer to this concept in many of our future discussions on creating sustainable systems.
Our final main concept is Equilibrium, which is defined as the state toward which a system will change in the absence of constraints. At the macroscopic scale, a system in equilibrium may appear static as a result of balance between forward and backward energy and matter flows. Systems that are not in equilibrium are either sitting in Metastable states or are altogether Unstable (transient) and in the process of moving toward equilibrium.