
River Processes
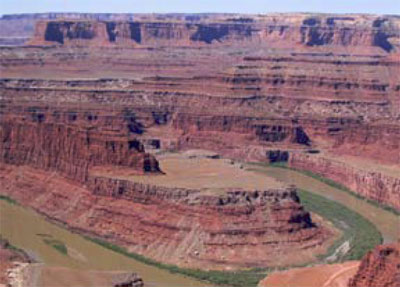
Southwest of Moab, Utah lies Canyonlands National Park. A “rough” park with few services available, little water (except for the rather muddy rivers), pot-holed roads, and awesome mountain biking, Canyonlands preserves the confluence of the Colorado and the Green Rivers. Many “visitors” to the park never actually enter it, choosing to gaze down on the Colorado from the vantage point at Dead Horse Point State Park. The two rivers of the park are incised a third of a mile (half a kilometer) or more into the red sedimentary rocks of the Colorado Plateau. Those rocks, mostly sandstones (made from sand) and shales (mud rocks, made from pieces smaller than sand), give the distinctive cliff-slope pattern of the canyons—resistant sandstones form cliffs and cap the flat-topped mesas, while softer shales form slopes.
The great Colorado Plateau, flanked by the spreading regions of the Basin and Range to the West, and the Rio Grande Rift to the east, occupies large parts of Utah and Arizona plus some of Colorado and New Mexico, and includes Zion, Bryce, Capitol Reef, Arches, Grand Canyon, Petrified Forest and Mesa Verde National Parks as well as Canyonlands, and many national monuments and other public treasures. The Colorado Plateau is noted for reddish, flat-lying, rocks from the Paleozoic of a few hundred million years ago.
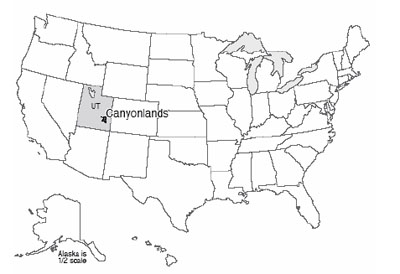
The details are not all known of how the Colorado Plateau avoided extreme deformation for hundreds of millions of years, while most of the rest of the west was being bent, broken, or erupted. The silica-rich continental rocks of the Plateau may be a bit thicker than in its surroundings, so the spreading of Death Valley was unable to tear the Plateau apart and instead jumped across to the east side of the Plateau to continue as the Rio Grande Rift (the valley in which the Rio Grande flows). The spreading even seems to have nibbled away at the Plateau, with a few big pull-apart, Death-Valley-type faults visible in places such as Red Canyon just west of Bryce (see the picture below). However, our concern here is slightly different— the role of streams.
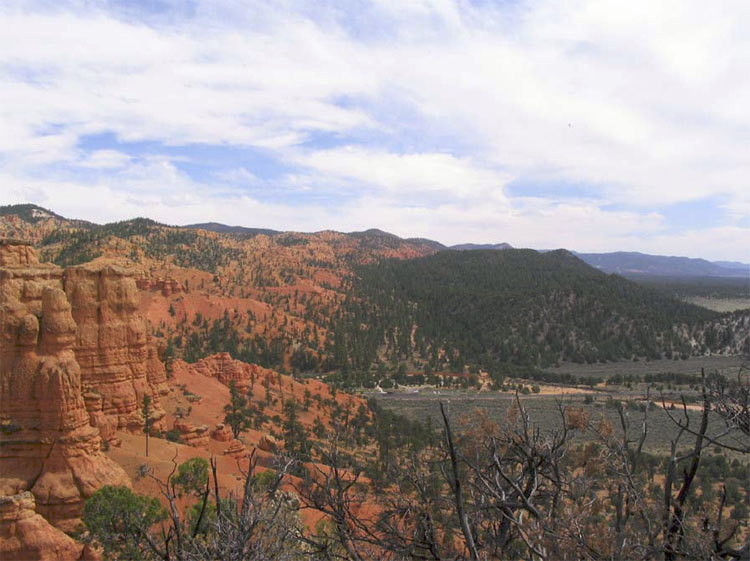
Rain, Rocks, and Rivers
The rivers of the Colorado Plateau are nearly as well known as their parks, for great rafting, incredible scenic views, and deep canyons. The Colorado, the Green, the San Juan, the Fremont, the Virgin and others have taken their place in history. But what are those rivers doing down there in the canyons?
Simply put, a stream or river is a conduit to take excess water, and sediment, from high places to low ones and usually to the ocean. Looking first at the water, rain or snow falls on the ground. Averaged around the world, rainfall (plus snowfall after it melts) is about 3 feet per year. Pennsylvania's annual rainfall is also right near the global average, as is much of the tree-covered eastern US. Some of this water evaporates directly, but most is used by plants and then is transpired (evaporates) from them. The evaporation from plants and from other surfaces usually is lumped together and called “evapotranspiration.” In a humid temperate climate such as central Pennsylvania, roughly two-thirds of the rainfall is involved in evapotranspiration and returned directly to the sky; in dry climates, a larger fraction of the rainfall—maybe almost all of whatever rain falls—may be returned to the air by evapotranspiration.
Of the water that avoids evapotranspiration, a little actually falls on lakes or streams, and some may fall on the land surface and then flow directly and immediately over the surface into lakes or streams, especially from the surfaces that humans have covered with buildings, roads, and parking lots, thus keeping the rain from soaking in. But most of the rain that avoids evapotranspiration soaks into the ground to form groundwater.
Soils and most rocks include interconnected spaces, either gaps between grains of sand, the cracks in the rock called joints, caves, or other openings. The ground acts a bit like a sponge, with water soaking in and then slowly draining out to the rivers. We will discuss this groundwater flow a bit more when we visit Mammoth Cave, next; for now, simply note that because gravity pulls water down, rocks near the surface usually have some air in the spaces even where conditions are damp, and deeper rocks usually have all their spaces filled with water. The surface separating the rocks with water-filled spaces from those containing some air is called the water table, and where the water table intersects the surface of the Earth, a stream or lake occurs.
Rivers flow even when it isn't raining because water is slowly draining through the ground from beneath hills to the rivers. The water table rises in elevation during wet times as the “sponge” of the Earth fills up with rain, and the water table falls during dry times as the sponge drains to keep the streams flowing. And, the water table is just below the surface in valleys, actually hits the surface at streams, but you must drill deeper under ridges to penetrate the water table and complete a water well.
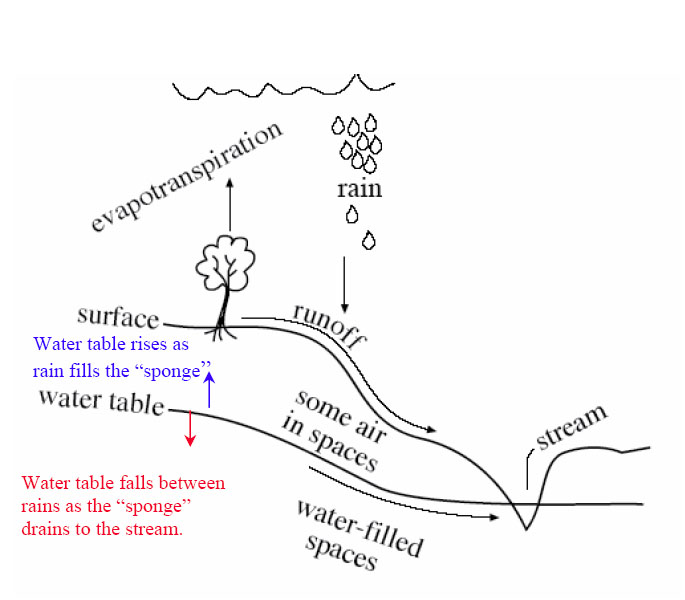
As we saw back at the Badlands, weather attacks rocks to produce loose pieces through the processes of weathering. And as we saw at the Gros Ventre slide in the Tetons, processes on hillslopes including soil creep and landslides deliver the loose pieces (which we can call sediment) to rivers. A river is then faced with a balancing act; it must transport both the water and the sediment delivered to it.
You may be able to think of many ways for the river to adjust if more or less water, more or less sediment, or bigger or smaller or “stickier” pieces (those more likely to clump together) of sediment are delivered. For example, if more sediment is delivered to a river than it can remove, then the sediment will pile up, raising the elevation of the bed of the river. This steepens the river—the elevation of the ocean where the river ends has not changed, but the elevation of the river bed away from the ocean is now higher—so the river flows faster and is better able to move the sediment. Deliver more water and less sediment, and the river will wash away all the sediment and have energy left over to carve into the river bed. This cutting downward will make the river less steep—because the river ends at sea level and can’t lower that, lowering the upstream reaches of the river must make the slope to the ocean less steep. A less-steep river will carry less sediment and so quit cutting its bed—the river tends to reach a balance in which it just removes the water and sediment supplied to it. In the process of reaching this balance, rivers also may adjust the width and depth of their channels as well as the steepness.
Rivers are diverse; a white-water rafter on the Youghiogheny River sees a very different setting than greets a river passenger on the Mississippi River going to New Orleans! Of the many river patterns, we often focus on two: meandering and braided (the Mississippi is a meandering river; the "Yough" has some meanders, but not nearly so well developed as the Mississippi). Whether a river meanders or is braided depends on the sediment supplied to the river as well as the water.
A river moves small sediment particles up in the water (suspended load), and larger pieces by rolling or bouncing them along the bed (bed load). If the river receives mostly small particles—what geologists call "fine-grained sediment"—the river typically will form a deep channel. Such a channel is especially efficient at transporting water, and the sediment in the water, because most of the flow is kept away from the river bed and river banks where friction with tree roots and other things slows the water. Very fine sediment—clay—can make fairly deep and steep river banks without collapsing, because the clay particles stick to each other—if you’ve ever worked with clay in art class, you know how well the clay particles stick together, and how much easier it is to make a pot out of clay than out of gravel or sand!
Such deep streams typically curve back and forth, or meander, along their paths. Put a tree’s roots in the way of the stream, and the water flow will curve around the obstacle. In doing so, the stream will race along the outside of the curve and erode it, and a meander bend will grow. (Meandering has been observed to occur without any tree roots in the way, and so is a bit more complex than just curving around obstacles.) Meandering streams usually occur in relatively flat, lowland regions towards the coast.
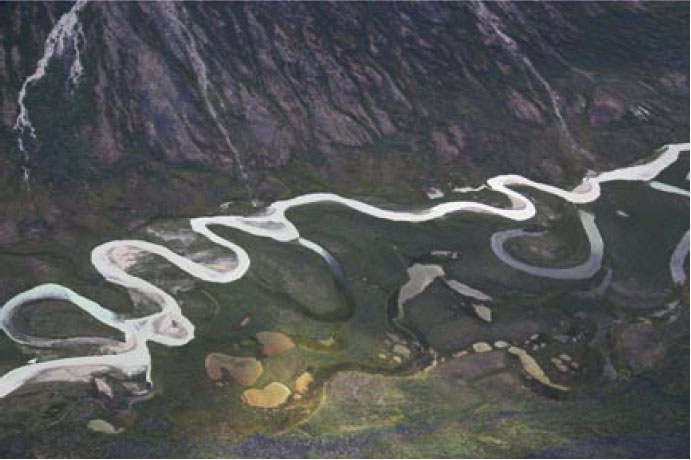
If a stream receives lots of sand and gravel or even bigger chunks, the large blocks will tend to plug, or dam, a single, deep and narrow channel. The stream then assumes a wide, shallow pattern that is efficient at rolling debris. When sand and gravel get really wet along a river bank, they cannot form steep slopes, as we saw with the mass movements in the Tetons, and the collapse of any steep slopes that start to form along a river bank contributes to having a wide, shallow stream. Within this broad stream bed, gravel (or boulder) bars often form when floods are ending and losing their ability to roll lots of sediment; the water then must flow around these bars. The splitting and rejoining of channels around bars, when viewed from above, looks something like ropes of water that have been braided together, so these are called braided rivers. They are common in upland regions, where steep mountain slopes shed landslides of coarse rocks into the channels.
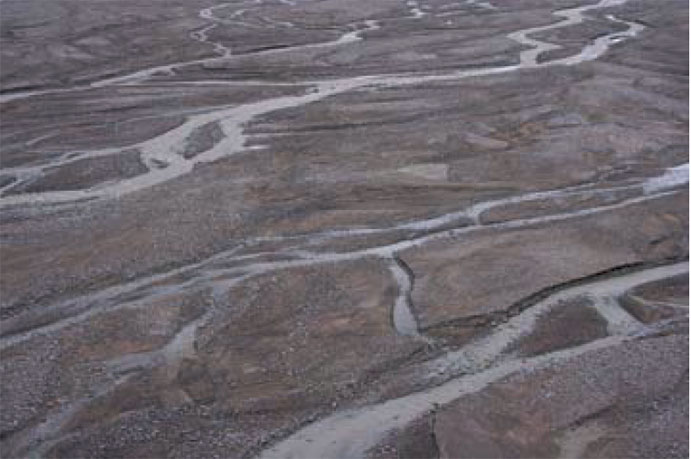
Again, please recognize that this is a very simple view of stream processes. You can find meandering streams in the flat bottoms of valleys in which beaver dams have trapped mud, for example. Streams with bedrock rather than sediment in their beds often have relatively straight single channels, as do many small streams with sediment beds. The key thing to remember about a stream is that it exists to move sediment as well as water.
A tremendous example occurs a little ways downstream of Canyonlands and upstream of the Grand Canyon. The Glen Canyon Dam was built on the Colorado River in the 1960s. The dam stopped floods coming through Canyonlands from reaching the Grand Canyon—water from floods that had raged through the canyon now is stored in the reservoir and then released gradually. Several things began happening to the river once the dam was completed. The dam trapped the sediment carried by the river, and released clean water, so the reservoir is filling with sediment, and in a few centuries or less will be full. Unique fish species that thrived in the muddy waters of the Grand Canyon suddenly were easy prey for clear-water species that were introduced, so many of the native species are endangered and disappearing now.
With no floods raging down the canyon, high water no longer piled up sand in corners of the canyon to make sand bars. The clean water was able to carry some sand and smaller pieces of sediment, so the existing sand bars below the dam were slowly washed away. The many types of wildlife that depend on sand bars thus were harmed—cottonwood trees that rooted in the sand, birds that lived in the cottonwoods, deer that came down to drink from the river by standing on the sand bars rather than the rocky cliffs. Floods on un-dammed side streams continued to dump large rocks into the Colorado, but the Colorado lacked the high flows to move this material onward, so the rapids at the mouths of side canyons began to steepen.
In the spring of 1996, an artificial flood was released from the dam to attempt to re-balance the system. Indications are that this flood was a partly successful experiment, rolling some of the big rocks out of the rapids at the mouths of the side streams, freeing sand trapped beneath, and forming that sand into bars. But, those bars weren’t very big and didn’t last very long. Additional human-made floods were released in 2004 and 2008, timed to occur when natural floods coming down side streams were delivering additional sediment, to help make bigger sand bars. Additional attempts may be made. Such human-caused floods cost money (lost hydroelectric power when extra water is routed around the hydroelectric plant in the dam to get water into the river in a hurry) and require lots of planning (you need to warn people camping or hiking in the Canyon before you suddenly flood them out!), and they can never get the Canyon back the way it was originally, but the artificial floods do seem to have helped restore some of the sandbars that are so critical for wildlife.
Meanwhile, upstream of the dam, as sediment builds up to fill the reservoir, sediment will also accumulate along the river upstream of the reservoir. There aren’t many people living there, but if there were, their fields and houses would begin to be buried by mud. A river slows down as it enters a reservoir, or any other lake or the ocean, and sediment is dropped from the slowing water. Unless strong waves and currents in the reservoir or ocean take that sediment away, a pile called a delta forms. But, the delta cannot be perfectly flat on top. If it were, then the stream would drop its load when it hit the flat spot and slowed down, and that would raise the flat spot. So, as the delta grows into the lake, the upstream end of the delta must build up so that the river still flows downhill, and that, in turn, will cause sediment to build up for some distance upriver (see the figure below).
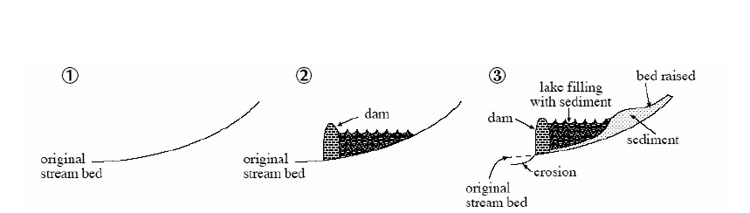
The troubles with the dam on the Colorado at Glen Canyon are not unique. Reservoirs immediately start filling with sediment, and so cannot last forever. This filling contributes to deposition of sediment upstream, which may bury houses or fields. The clean water released tends to pick up fine-grained sediment below the dam, but the lack of floods means that the river is no longer able to move coarse-grained sediment. Hence, the river bed is changed, affecting species that lived there. The lack of floods often has huge effects on the plants and animals that live along the river; what had been a region reserved for wildlife adapted to the changing water levels and channels becomes a place for humans to settle in the shadow of the dam.
When two dams were built on the Elwha River, which flows north from Olympic National Park, the dams contributed to blocking salmon runs, to washing away the sand and gravel in which the salmon had spawned downstream of the dam sites, and to turning an annual “flood” of 300,000 salmon into a trickle of barely 3,000 salmon. (A fish hatchery was built instead of fish ladders around the first dam, but the hatchery was quickly abandoned.) Once the spawning bars were washed away below the dam, the river quit delivering sediment to the beaches of the Strait of Juan de Fuca (an arm of the Pacific Ocean); as the beaches washed away, the native peoples were no longer able to carry on their traditional shell-fishing, and engineering was required to protect the nearby harbor of Port Angeles, which was no longer guarded by sediment-fed bars. In an ambitious plan to help the beaches, the river, the salmon and the park, the federal government purchased the dams. One was removed in spring of 2012, with the second slated to follow quickly. The river, beaches, harbor and more will take a while to get back to "normal," because so much was changed by the dams, but there is much optimism about the recovery. You can read more, and track the progress, at the official website for Olympic National Park.