
8.1 Renewable Energy Basics
Renewable energy has been a catchphrase of recent decades. It has been both the subject of government policies and extensive research. We are searching for efficient ways to move from the easily accessible but finite fossil fuel energy to other types of energy available on earth that are either unlimited or can be replenished within a much shorter timescale.
We have one lesson to review this topic. This does not allow us to go anywhere in depth while discussing specific technologies, but we will take some time to consider a few examples of how the renewable energy technologies are chosen, implemented, and assessed from the sustainability standpoint.
The term renewable essentially means that the energy source is not exhaustible on a human timescale. This, however, does not always mean that it has infinite capacity or constancy. We will see that different sources of renewable energy have different limitations, which must be taken into account when tuning the technologies to a particular application and locale.
The main types of renewable energy resources are schematically summarized below:
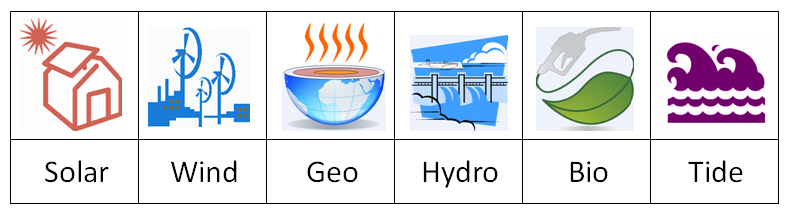
The technologies that are designed to convert the above kinds of available natural energy to usable power or heat are expected to play significant roles in future energy economy. However, markets involving those technologies are still developing, and at the moment it is not so easy to predict which of them will become most prominent.
I am sure you are somewhat familiar with the operating principles of these technological systems. You may be still curious to look through the Renewable Energy Wikipedia article to refresh your basic knowledge on those types of energy conversion. Consider this as an optional resource unless you feel you have serious background gaps to fill.
Because we will be going through some numerical examples involving energy, it would be also useful to have a refresher on terms and units.
Quick refresher on energy terms and units
Energy is the capacity of a system to perform work. Energy is also an extensive quantity, measured by amount. Thus, you need a certain amount of energy to perform a certain work. Energy can be added, subtracted, converted from one form to another, but it cannot be created or destroyed according to energy conservation law.
Units to measure energy:
- Joule (J) - International SI system. Convention: 1 J is the amount of energy necessary to move an object over 1 meter against the force of 1 newton.
Joule = Newton x meter = Pascal x meter3 = Watt x second - British Thermal Units (btu) - used in fuel and thermal applications. Convention: 1 btu is the amount of energy necessary to heat 1 pound of water by 1 degree Fahrenheit. 1 btu = 1055 J
- Kilowatt-hours (kWh) - used in electricity. Convention: 1 kWh is the amount of energy being transmitted with the power of 1 kW over 1 hour
1 kWh = 3600 kJ
Power is the amount of work performed per unit of time. Power can be understood as the rate of energy conversion.
Units to measure power:
Watt (W) = Joule / second
Often, the energy converting systems are rated by power.
For example:
40 W light bulb - it means it consumes 40 Joules of electric energy per second;
250 W solar panel - it produces 250 joules of electric energy per second (under standard conditions), which converts to 6 kWh per day;
20 MW wind farm - it generates 20 MJ of electric energy per second or 20 MWh per hour (at maximum operation load).
Role of energy technologies in sustainability
Since energy is the main commodity ensuring well-being and sustainability of human society, the role of energy technologies is critical. The efficiency with which we can convert and distribute energy essentially determines our standard of living. Speaking the systems language, sustainability would require maintaining the stock of usable energy at the demand level. Consider the system diagram in Figure 8.2.
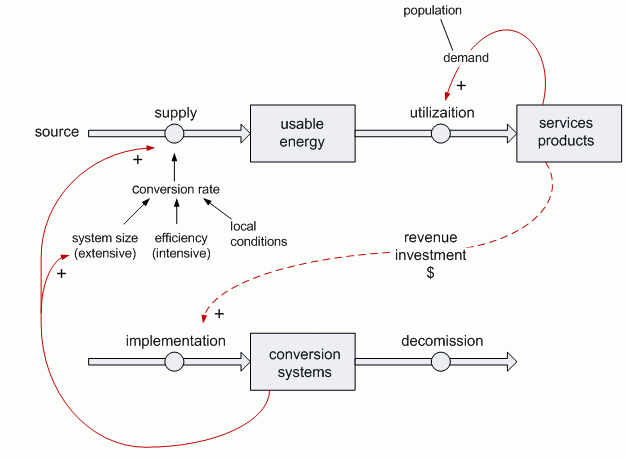
The main goal of the sustainability system shown above is to keep up the usable energy stock, as it is closely connected to the amount of demanded services and products for society. These two stocks are related through the energy utilization rate.
To make sure the society demand is met, the energy supply rate (left-hand valve) should match the energy utilization rate (right-hand valve). It is this supply valve where energy conversion technologies play the major role. The energy is supplied from a source (which we can assume to be unlimited, e.g., sun energy); however, the conversion rate of the available energy from the source to the usable energy will be the main limiting factor. Conversion rate will depend on technology efficiency, system size, and will be affected by environmental conditions at a particular locale.
So, what can be done to maximize the energy conversion rate at the supply valve?
- Maximize efficiency. This depends on the stage of the technology development, physics of the process, materials, design, and other things that may be improved through research and development efforts.
- Scale-up the conversion system. Feasibility of this measure may be limited by technology cost and available space or land area (to accommodate the equipment, etc.)
- Tune technology to local source and conditions. Local conditions will dictate the adequate choice of technology to employ (obviously, solar energy is great where there is a lot of sun, and wind energy option would be suitable to the places that are windy). Also, system design should be adapted to the geometry and timing of the natural resource – such supplemental technologies as tracking and energy storage help increase the overall conversion.
All the above factors shown on the diagram will affect the energy conversion rate.
While we can identify the factors that maximize the conversion and enhance the system’s function, the system may be not resilient until there is a return balancing loop. One of such loops (shown by dashed line on the system diagram in Figure 8.2) shows the investment of the energy generation revenue into creation of new energy conversion systems. This feedback has been more strongly established over the past decade, but it can be also driven to one side or the other by government incentives and social factors. Furthermore, wider implementation of the renewable energy conversion systems will result in an increase of renewable energy supply and higher energy stock for consumers.
Establishment of this system will eventually ensure renewable energy economy; however, this process is still hampered by strong economic competition from the non-renewable energy worldwide, which is currently in a dominating position by scale, profit, and infrastructure development in a number of major players - e.g. China, United States, Russia.
This is only one slice of the quite complex “tug-of-war” issue of commercialization of the renewable energy. It is anticipated that recent advances in research and development will be able to increase the energy conversion efficiency and thereby further upgrade its market value. The technical status of several prospective energy technologies is reviewed in the following sections.