
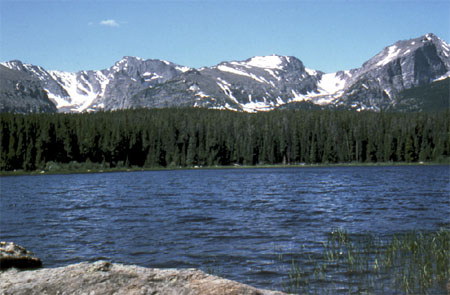
Mountain Building and Metamorphism
Rising high above Estes Park, Colorado, and almost within shouting distance of the population centers of Boulder and Denver, Rocky Mountain National Park is a natural destination for the crowds that throng to this mountain playground. Long’s Peak, at 14,256 feet (about 4300 m), dominates the south-central part of the park; the peak was first climbed in 1868, by a party that included John Wesley Powell, the man who later commanded the first boat passage of the Grand Canyon and then led the United States Geological Survey. Numerous peaks over 13,000 feet (4000 meters) in Rocky Mountain lure climbers.
Small and rapidly shrinking active glaciers still carve the mountains, and much greater glaciers of the past left the numerous tarn lakes, moraines, and other features that decorate the park. Trail Ridge Road surmounts the high tundra of the park, giving the visitor a first-hand look at periglacial processes and ecosystems (those of cold regions; more on this later). The Colorado River rises on the west slopes of the park, and lovely little trout streams such as the St. Vrain flow down the east slope. Bighorn sheep and elk attract traffic jams in Horseshoe Park.
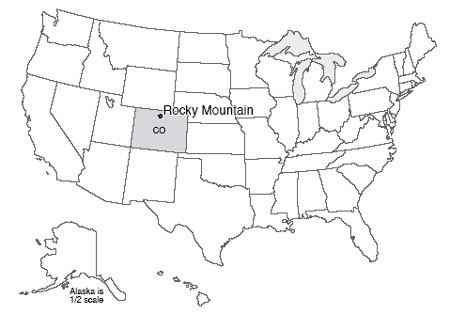
It is a tad embarrassing to say that we don’t fully understand the Rockies yet, including those of Rocky Mountain National Park. Oh, the long history of mountain building, erosion, glaciation, etc., is well-known—we can tell the story. But most mountain ranges hug coasts, or are trapped inland only by the destruction of the ocean to which they once were coastal, whereas the Front Range of the Rockies is as far as almost 1,000 miles (1600 km) from the coast, yet the Rockies are not the direct result of obduction.
The U.S. West is a complicated region (see the Enrichment section for a little more on this). The continent has been approaching and overriding the East Pacific Rise spreading ridge. The San Andreas Fault is the product of the rise running into the trench. Before these met, subduction had been occurring from push-together motion, but with a little slide-past motion thrown in. After the meeting, the subduction stopped, so the push-together stopped, but the slide-past remained to make the San Andreas. To the north of the San Andreas Fault, subduction is still active, forming the Cascades including Mt. Rainier and Mt. St. Helens.
Long ago, the west-coast subduction zone started in the usual way, with old, cold ocean floor going down into the deep mantle. But as the continent approached the spreading center, the down-going ocean floor became progressively warmer and more buoyant. The ocean floor didn’t “want” to go down, but it was still attached to the older, colder floor ahead of it that was going down. So, the ocean floor went under the continent, but stayed high rather than sinking, and rubbed along the bottom of the crustal rocks rather than plunging steeply into the mantle. The friction between this buoyant subducted ocean floor and the crust above, in turn, caused thrust-faulting and crustal thickening far inland (see the figure just below). Because the western part of the country has been built up of many old rock bodies and sediment piles bulldozed from the Pacific, there are scars of many old faults and other geological features that have been reactivated by the recent events, so mountains and valleys have formed along the old weaknesses in response to the new pushes.

As to exactly how this came to produce 14,000-foot (4300-m) peaks in the Rockies, geologists can tell the story, but it isn’t clear that any geologist could have predicted this story without seeing the rocks first. Science moves from explanatory (easier) to predictive (harder), so we still have some work to do. (And, we've oversimplified a bit here; see the Enrichment for more.)
If you drive west from Boulder up the slope to Rocky Mountain National Park, you will go through sedimentary rocks, made from sediments brought down from the current Rockies and from earlier versions of the Rockies. As folding and faulting pushed up the mountains farther west, and as erosion of those peaks allowed “bobbing up” of their thickened deep root, the sedimentary rock layers were tilted (see the figure below), so you actually will be driving into older and older rocks as you go. Eventually, you will reach the heart of an old (Precambrian) mountain range that also forms the heart of the modern one. Even to a casual observer, the rocks here have been “beaten up.” Follow a single layer in a rock, and you will see that the layer twists and bends, doubles back on itself, or even pinches out in places. Analyze the rocks chemically, and you will find a composition similar to the common sedimentary “mud” rock called shale, but the rock clearly is not shale.
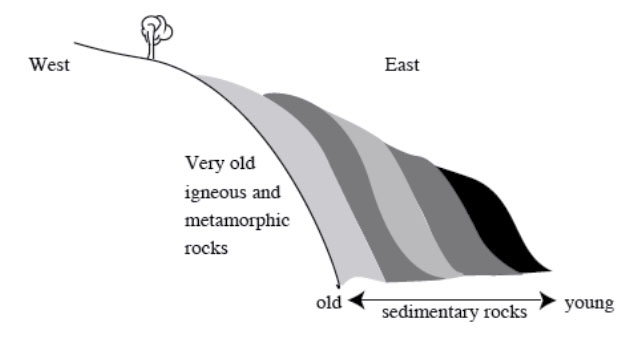
Cooking the Earth
Think about cooking. If you mix up a bunch of ingredients to make cake batter, throw the mixture into a pan and put it into a warm oven, the cake you obtain will not be very similar to the mixture you started with. Grill a steak, and the original cow part will come out quite different. Marinade the steak before grilling, and more differences appear. It is common knowledge that a material that is stable in one environment will change if it is placed in a different environment. This is true of everything (and everyone!) on Earth.
The Earth clearly has a great range of conditions. The inside of a mountain range is hotter, higher-pressure, and less affected by acidic groundwaters than is the surface. Materials that are stable at the Earth’s surface (such as the clays in a piece of shale) are not stable deep in a mountain range. The minerals change, grow, and produce new types even without melting. This process is called metamorphism. Metamorphism makes rocks that many people consider to be especially pretty, produces some wonderful gems, and contributes rock names that make good puns. (The Geoclub at Wisconsin used a metamorphic rock, a volcanic rock, and a sedimentary rock in claiming that geologists are “gneiss, tuff, and a little wacke.”) You can read a little more about rocks and minerals in the Enrichment section.
Where Tectonics Meet People: Tsunamis
We’ve been looking at the ways the planet moves rocks around and makes mountains, and some of the ways that mountain-building can be dangerous to humans. Volcanoes and earthquakes are sometimes truly dangerous and damaging. But it is worth remembering that, in the developed world, only about 1% of us die in “accidents,” and car crashes greatly dominate those deaths (so 99% of us die of other things, such as heart disease, cancer, etc.). With good scientific warnings, good zoning codes, trained medical personnel, hospitals and ambulances to take care of us, nature just doesn’t kill that many of us. (In the less-developed world, this is, sadly, less true.) For the developed world, things we do to ourselves (smoking, eating and drinking too much, not exercising enough) are far, far more destructive of health and life than anything the planet does to us.
But, it is still wise to know about the dangers from the Earth. And now that we’ve completed the tour of mountain-building, we will look at another hazard. Tsunamis are not directly related either to the Great Smokies or the Rockies, but anything that makes earthquakes, volcanoes, or really steep slopes in or near the sea might be involved in a tsunami. And tsunamis can be truly horrific. We’ll start with a surprising hot-spot tsunami, and then look at some others.
On the flanks of many of the Hawaiian Islands, including Lanai, Molokai, and Maui, to at least 1,600 feet (500 m) in elevation, there are deposits of broken-up, mixed-up, battered corals, other shells, and beach rocks. Corals are undersea creatures, and surely don’t grow 1,600 feet above sea level. Some corals grow just below sea level and later are raised above the water by mountain-building processes; however, these Hawaiian deposits occur on islands that are sinking as they slide off the “hill” made by the rising motion of the hot rock of the Hawaiian hot spot, and the deposits are geologically too young to have been raised so far by mountain-building processes. Clearly, something strange happened.
One of the deposits in particular is the same age as a nearby, giant underwater landslide, as nearly as the age can be measured, which may suggest something. The Hawaiian volcanoes have rather gradual slopes above the water, where the hot, low-silica lavas spread out to make shield volcanoes. But when lava hits the water, the hot flows cool and freeze very quickly, and can make steep piles. Too steep, and eventually the side of the island fails in a great landslide, perhaps when melted rock is moving up in the center of the island and shoving the sides out to make them steeper. Surveys with side-scanning sonar have shown where several such slides have slipped. (Try saying the previous sentence five times fast!) Such slides can be miles thick, tens of miles wide, and over 100 miles long.
Now, if a chunk of rock miles thick and tens of miles wide suddenly starts moving, maybe at hundreds of miles per hour, it will shove a LOT of water out of the way. Where will the water go? The answer is that it will make a huge wave, or tsunami, that will race up any land it encounters after crossing the ocean. Imagine a wave that would run far inland and reach heights of 1,600 feet above sea level. Fortunately, the highest deposits in Hawaii are from a tsunami about 110,000 years ago, long before people were in the way there. Although many such tsunami-generating landslides have occurred, they typically are spaced thousands of years apart or more. But, we can’t absolutely guarantee that there won’t be another one.
The word tsunami comes from two Japanese words, for harbor and wave, a sort of shorthand for a wave that devastates a harbor. Most tsunamis are generated by undersea earthquakes, but undersea landslides or volcanic eruptions, and even meteorite impacts in the water, can generate tsunamis.
Tsunamis move rapidly across the deep ocean, with speeds of 300 to 500 miles per hour (600 to 1000 kilometers per hour). In the deep ocean, the “bump” of water that is the wave of a big tsunami may be only a very few feet high, but may extend well over 100 miles in the direction it is moving. Waves slow down as they enter shallower water, and the leading edge of a wave hits shallow water before the trailing edge. So, the leading edge slows as it nears the coast, the trailing edge catches up, and the wave goes from being long and low to being squashed and high. Even so, the tsunami wave is usually not a towering wall of water, but a strong surge, something like the tide coming in but higher (hence the mistaken name “tidal wave”).
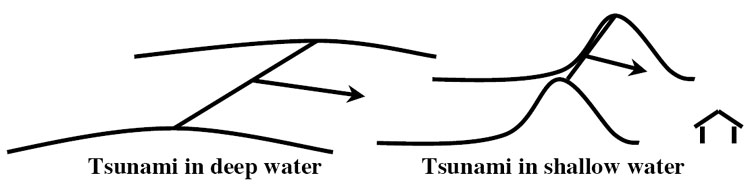
An especially nasty feature of a tsunami is that the water often goes out before it comes in (waves consist of troughs and crests, and some places get the crest first while other places get the trough first). The sudden retreat of water and exposure of the sea floor tempts people to walk out and look around. Then, the ocean returns faster than a person can run. The outcome is predictable and unpleasant.
Terrible tsunamis have occurred. Still horribly fresh in our memories is the Indian Ocean tsunami of 2004, which was triggered by the second-largest earthquake ever recorded, and which killed over 300,000 people. Japan was much better prepared for the 2011 Tohoku earthquake, but almost 16,000 people still died, mostly from the tsunami. The massive 1883 explosion of the volcano Krakatau in Indonesia essentially destroyed the island, with tsunami waves observed as far away as England. Floods raced miles inland on Java and Sumatra, with probably tens of thousands of deaths. Another volcanic eruption, likely in the 1600s BC, of the Greek island volcano Santorini, pushed a tsunami perhaps 300 feet (100 m) or more high across the coast of Crete, and may have contributed to eventual demise of the Minion civilization there. Many commentators have suggested that this is the source of the myth of Atlantis. The great 1964 Alaska earthquake generated a deadly tsunami that killed 118 people, with deaths as far away as California. In 1958, an earthquake-caused landslide in Lituya Bay, Alaska, caused a tsunami that included a wave 50-100 feet high out in the bay, which a father and son safely rode out in a boat. They watched in awe as the wave then ran 1,800 feet up an adjacent coast; five people were killed in the event.
There isn’t a whole lot that can be done to stop tsunamis, but loss of life and property damage can be limited. Tsunami warning systems are functioning in many places, and are being extended rapidly. When instruments (called seismometers) sense the shaking of the Earth from a large undersea earthquake, volcano, or other disturbance, if characteristics suggest that a tsunami is likely, communications are sent out to various agencies concerned with safety, and sirens or other warnings on beaches are activated to get people away from the coast before the tsunami arrives.
The Indian Ocean tsunami seems to have been especially deadly in places where human activities had caused damage to the coral reefs and coastal vegetation that would have blunted the strength of the wave, so maintenance of these natural buffers can help the people living there. And, scientists can figure out where tsunamis are likely, how big and how frequent they are likely to be, and then zoning codes can be enforced so that people build in appropriately safe ways on appropriately safe land if they want to live in an area.