
Additional reading from www.astronomynotes.com
So far, we have discussed the origin of the Universe and the age of the Universe, but not its ultimate fate. This has been a question that has been pursued for many years, and a number of theorists were considering possible ideas for the fate of the Universe concurrently with the development of the Big Bang model. If you compare college astronomy textbooks today to those published about 20 years ago or more, though, you will find that this part of the discussion of cosmology has changed substantially since about 1998. The reason is because newly discovered evidence for dark energy complicates the matter. Dark matter plays a role in determining the fate of the Universe, too.
We have already encountered dark matter during our discussion of the rotation curve of the Milky Way, but I will go into some more detail here. Recall that in the Milky Way, we find that the outer parts of the Galaxy are rotating much faster than expected if all the matter in the Galaxy is visible matter. Based on the rotation curve of the Milky Way, it appears that the Galaxy contains more dark matter than luminous matter. Beyond individual galaxies, though, there is also evidence for dark matter in clusters of galaxies. Just like the rotation curves of Galaxies, you can also study the velocities of galaxies inside massive galaxy clusters. The escape speed from an object (in this case a cluster) depends on the mass of that object. In many clusters, the velocities of the galaxies in that cluster suggest that the cluster could not remain bound if all it contains is normal matter. There must be additional dark matter in the cluster, or else many of the galaxies would escape.
Additional evidence for dark matter in galaxy clusters comes from images like the one below. When we observe some clusters, we see another effect predicted by Einstein, called strong gravitational lensing. Since Einstein predicted that massive objects can warp spacetime, he showed that the light from a background object will be bent if it passes by a massive object, like a galaxy cluster. Here is an image of a cluster lensing a background galaxy, just as predicted.
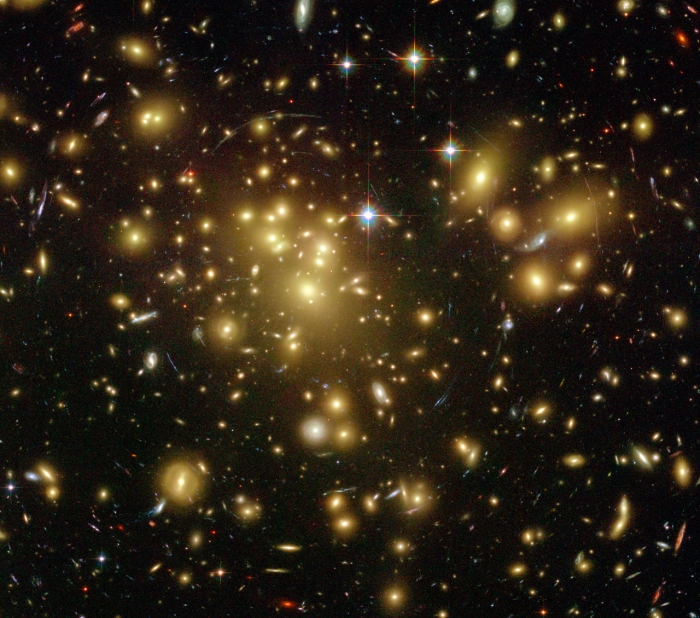
The arcs that you see in between the yellow galaxies are distorted images of the background galaxy. The details of the lensing effect depend on the mass of the lens (that is, the more massive the lens, the more distorted the background galaxy), and show that this particular cluster contains more mass than it appears based solely on the luminous galaxies. Like the case for individual galaxies, it appears that the amount of dark matter in clusters like this is significantly larger than the amount of luminous mass.
The evidence for dark energy comes from different sources. The first piece of evidence comes from the Hubble diagram as calibrated by Type Ia supernovae. These objects are so luminous that they allow us to measure their distances accurately out to redshifts of . When the first Hubble diagrams were constructed using distances obtained from Type Ia supernovae, though, astronomers found a significant deviation from expectations. The supernovae were systematically fainter than expected at large distances. Below is a Hubble Diagram using Type Ia supernovae studied by two different teams.
You can see in both the top panel and the bottom panel that the points with redshifts greater than about 0.5 seem to deviate from the straight line. This suggested that our (at the time) accepted models for the expansion of the Universe were incorrect.
We know that there is some matter in the universe (we live on a giant ball of matter called the Earth, after all), but our measurements of the luminous and dark matter in the Universe have shown that there is not enough matter to close the universe, or even to make it flat (that is ). In the mid-1990s, the data suggested that the universe is open and that the total amount of luminous matter plus dark matter in the universe was only about 30% of the critical amount necessary for a flat universe. Given that there is some matter in the Universe, though, we expected that for objects at large distances, their distances would deviate from Hubble's Law. The reason is that the combined gravitational pull of all of the objects on each other would oppose the expansion of the Universe, causing it to decelerate. Because of deceleration, at the largest distances, objects should appear closer to us than predicted by their redshift. So for many years, the question that many astronomers were pursuing using different research techniques was "How much is the universe decelerating?".
However, for supernovae, the exact opposite was found. These objects appear to be farther away from us than predicted by their redshifts and Hubble's Law. The only way for this to happen is if the expansion of the universe is accelerating, not decelerating. In order for the universe to accelerate, there must be some force pushing all of the galaxies away from each other, and this force must be strong enough to counteract the deceleration by gravity. Today, we do not know what is the exact cause of this force, just that it exists. Since we call the matter that we cannot observe directly "dark matter," we call this new mysterious force (or equivalently, the energy provided by this force) dark energy. If we add in the contribution of dark energy to the density of the universe, it appears that the combination of normal matter, dark matter, and dark energy is enough to make the universe flat ( , but here ). Remember, our measurements showed that matter makes up only about 30% of the critical density, so dark energy makes up the other 70%! It appears that the 30% matter is about 4% normal matter (people, planets, stars, galaxies) and about 26% is dark matter. So this means that at this time, astronomers can only directly observe about 4% of the universe, and the other 96% is divided up among this peculiar dark matter and dark energy, which we have still not identified.
You may think that this is a bold claim based solely upon the distances to a handful of Type Ia supernovae, but the fluctuations in the CMB seen by WMAP also predict that normal matter only makes up approximately 4% of the Universe. Thus, the results from WMAP appear to confirm the results from Type Ia supernovae. Below is an image from the WMAP team for their predictions for the contents of the Universe.
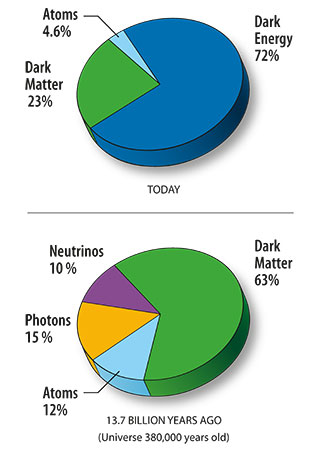
Type of Matter | Percentage |
---|---|
Atoms | 4.6% |
Dark Matter | 23% |
Dark Energy | 72% |
Type of Matter | Percentage |
---|---|
Neutrinos | 10% |
Atoms | 12% |
Photons | 15% |
Dark Matter | 63% |
At this point, let us reconsider the question of what will happen to the Universe over time. Right now, it is difficult to say, because we do not understand dark energy very well. However, it appears that, given the accelerating expansion of the Universe, the Universe will grow larger and larger and colder and colder. All of the luminous objects in the Universe will eventually die out, and the Universe will eventually end in a "Big Freeze," where it will be too cold to support any life.