
Finally, we come to the so-called General Circulation Models or GCMs. GCMs attempt to describe the full three-dimensional geometry of the atmosphere and other components of Earth's climate system. Atmospheric GCMs numerically solve the equations of physics (e.g., dynamics, thermodynamics, radiative transfer, etc.) and chemistry applied to the atmosphere and its constituent components, including the greenhouse gases. In more primitive GCMs (the earlier generation models), the role of the ocean was treated in a very basic way, e.g., as a simple slab of water where only the thermodynamic role of the ocean was accounted for.
Current generation climate models typically include an ocean that plays a far more active role in the climate system. The major current systems are modeled, as is their direct role in transporting heat poleward. When the dynamics of the ocean and its interactions with the atmosphere are explicitly resolved by a climate model, the model is referred to as Atmosphere-Ocean GCM, or AOGCM, or sometimes simply a coupled model. Most state-of-the-art climate modeling centers today run AOGCMs. In addition, many state-of-the-art climate models today include a detailed description of the hydrological cycle (which couples atmospheric, terrestrial, and ocean reservoirs of water and the flows between these reservoirs) as well as the role of terrestrial biosphere, the continental ice sheets, and even the ocean's carbon cycle and its interactions with the ocean and the atmosphere.
Unlike simpler climate models like EBMs, GCMs and AOGCMS can be used to study a variety of climate attributes other than surface temperature, such as atmospheric temperature profiles, rainfall, atmospheric circulation, ocean circulation, wind patterns, snow and ice distributions, and many other variables that are part of the global climate system.
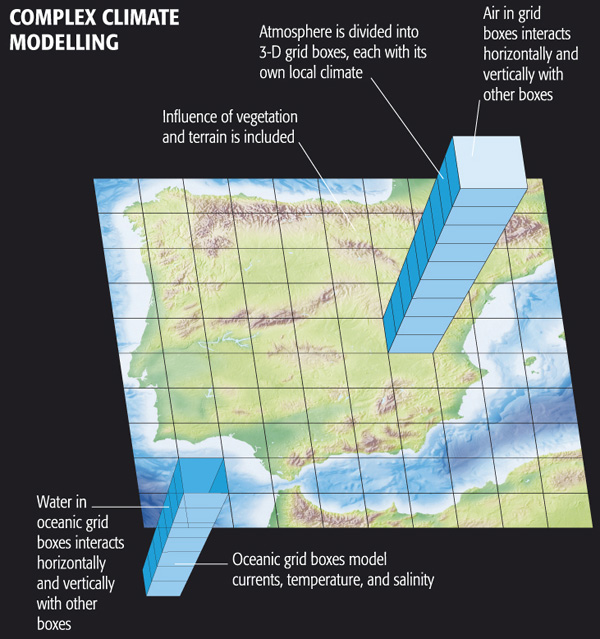
Complex Climate Modelling includes:
- Water in oceanic grid boxes interacts horizontally and vertically with other boxes
- Oceanic grid boxes model currents, temperature, and salinity
- Influence of vegetation and terrain is included
- Atmosphere is divided into 3-D grid boxes, each with its own local climate
- Air in grid boxes interacts horizontally and vertically with other boxes
© 2015 Dorling Kindersley Limited.
EdGCM
The EdGCM project, funded by the U.S. National Science Foundation and spearheaded by scientists associated with NASA Goddard Institute of Space Studies (GISS), uses the GCM originally used in a number of famous experiments (which we will review later in this lesson) by climate scientist James Hansen, Director of GISS. This model was developed in the 1980s and is primitive by modern standards, but it includes much of the important physics that is in current state-of-the-art climate models and it is far less computationally intensive. The scientists at EdGCM have ported the model into a format that can be run on a simple desktop or laptop computer (both PC and Mac). Originally it was free, but to cover expenses for the project, a minor fee is now required for download. Your course author has downloaded EdGCM onto his own laptop (MacBook Pro) and is now going to show you the results of several experiments he has run.
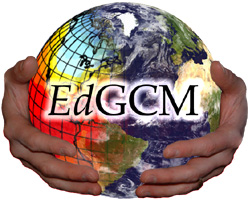
Video: EdGCM Demo - part 1 (3:47)
PRESENTER: OK, so we're going to run a GCM, a very famous GSM, in fact. This is the GCM that was constructed back in the mid-1980s, which was used for a number of very famous climate modeling experiments by James Hansen and his group at the NASA Goddard Institute for Space Studies that we'll be talking about a little bit more later on in this lesson. So that model has actually been taken and put in a format that can be run on a PC, on a Mac or a PC.
Of course by modern standards, the computational requirements of climate models written in the 1980s are far exceeded by current day state of the art models. But because they are a couple of decades old, they can in fact be run fairly efficiently now on simple laptops and PCs like we're going to do. Now, this model is available at the website EdGCM.com.
I would have had students in the class download the model and run it themselves. That was possible a few years ago. Unfortunately, now you actually have to pay to obtain the model. And so I will be doing some experiments with the model. And you will be watching me do these experiments rather than actually downloading it and running it yourself. But if you felt so motivated, you could indeed download this climate model and do the very same sorts of experiments that we're doing right here on your PC, or your Mac, or whatever computer you have.
So let's go ahead. I'm going to look at this Doubled CO2 experiment. If we click here, we can get some information about what that experiment is. It basically takes the CO2 concentration from 1958 and instantaneously doubles that CO2 concentration. And then we see how the climate model responds to that sudden increase in CO2.
Now, because of the presence of a large ocean that has large thermal capacity in this model, it takes quite a bit of time for the climate model to equilibrate to do that increase in CO2 concentrations, in fact, several decades to near a century. So this underscores the point that when we're looking at transient climate change, whether we're looking at observations are looking at the results of a climate model, we are in fact observing a system that is not in equilibrium. And that might take quite a bit of time to equilibrate to whatever change enforcing is imposed.
So ultimately, we know that this model will warm the amount that is consistent with the sensitivity of the model in response to that CO2 doubling. But it will not achieve that equilibrium warming for several decades, again, to nearly a century. And so what I'm going to do now is actually continue a run of that model that I started previously. And I'm just going to click on that. And I should be able to run.
Video: EdGCM Demo - part 2 (3:39)
PRESENTER: OK, so I'm running that experiment. It started in 1957. Then we instantaneously doubled the CO2 concentration. And now we're letting the model equilibrate to that instantaneously doubling of CO2.
And when it comes into equilibrium, it should have warmed by an amount that is consistent with the sensitivity of this particular model. And again, this is the NASA Goddard Institute for Space Studies climate model from the 1980s that was used in a series of famous experiments by James Hansen, by climate scientist James Hansen.
So we're running the model. I've been running this for several days, in fact. And as you can see, I'm now all the way up through September 2012. So we started out in December 1957. The model has now reached September 2012.
And as you can see, I get about one simulation day per second. So it takes the better part of a day running this climate model to simulate anything approaching a century, a long time period. But that's quite fast. By comparison, say, with where things stood in the 1980s when it would take that long to run a simulation like this on a state of the art computer, now we can run it on a desktop.
So we're letting the days tick off day by day. The model is solving all of the equations of motion. It's solving the governing equations of the atmosphere and the ocean. It's calculating the radiative fluxes, the incoming solar radiation. It's computing the distribution of infrared radiation within the Earth's system, the diffusion of heat into a mixed layer ocean.
It's solving the full set of governing three-dimensional equations that describes the coupled ocean atmosphere, cryosphere system. The ocean in this model is fairly simple by modern standards. Today climate models of this sort simulate the complex motions of the ocean currents.
This particular model treats the ocean simply as a slab of water with an appropriate amount of thermal inertia. It can absorb heat. It can give up heat to the atmosphere. It can respond to radiative imbalances.
There is a parameterization of heat transport. So the ocean transports a certain amount of heat poleward. As we know, it needs to. But that heat transport is fixed. It's not variable as it is in modern-day climate models which allow for changes in the intensity of the ocean currents.
So the ocean is pretty primitive by modern standards. And many of the components of this model are in fact primitive by the standards of state of the art models today. But as we'll see, this model is sophisticated enough to have made some surprisingly accurate predictions.
OK, so we're closing in on the end of 2012. And what I'll do is I'll let this model run out to the end of 2012 so I have one more complete year of data. And then we'll start looking at the output of this climate model simulation.
Video: EdGCM Demo - part 3 (2:14)
PRESENTER: OK so let's look at the simulation results. And by the way, there are a number of experiments that can be performed using EdGCM. One of them is the doubled CO2 experiment that we just talked about.
And you can see how there are various settings. You can tell the model what component, what ocean model to use, a mixed layer model with a parameterization of heat fluxes, or a simpler model if you choose to do so. You can change how vegetation and topography is parameterized. So there are lots of different settings in the model that one can change.
And there are various presort of determined experiments that you can just run off of the main menu in EdGCM. But if you liked, you could design your own experiment. You could set all these settings to use that particular version of the model that you want to use. You could specify exactly how to change forcings, whether they be natural forcings like CO2 or other anthropogenic forcings, methane, and CFCs.
You can change natural forcings like solar output or even the Earth orbital geometry changes that we know are important on very long time scales. So there are a variety of experiments that you can perform. And a number of the sort of predetermined experiments are indicated here.
You can simulate the last Ice Age. You can simulate Snowball Earth. We've alluded to the fact that in the past there were periods in Earth's evolution where we believe Earth was entirely frozen. And you can do that Snowball Earth experiment With EdGCM.
But we're going to continue to analyze this CO2 doubling experiment that we were running. And we will look at the output now. As you can see here-- well, we'll do that in a moment.
Video: EdGCM Demo - part 4 (1:57)
PRESENTER: OK, so unfortunately there are quite a few bugs still in EdGCM. And sometimes it freezes up and you have to restart it, which I've done here. So forgive the lack of continuity. But we're going to pick up where we left off. So we have the [INAUDIBLE] doubling experiment. As you can see, we now have years 1958 through 2012 completed. So we've got more than 50 years of output of this [INAUDIBLE] doubling experiment. And this most recent year, 2012, you saw me complete it a little bit earlier.
So we will extract the time series, various variables. You can see that I've selected precipitation, surface air temperature, ocean ice cover, snow cover, water content of the atmosphere, sea surface temperature, and the ground albedo. Or if you like, we could select instead the planetary albedo, which would include both the ground and, for example, cloud albedo. So I've selected these variables.
And now what I'm going to do is generate time series, annual averages for each of these variables for each of the years of the simulation. So I'll be able to plot out time series of the various quantities. And you can see it calculating the annual averages right now. It's going through each month of each year and calculating an annual average. So we'll let it finish that process. And then we will look at the output shortly.
Video: EdGCM Demo - part 5 (4:12)
PRESENTER: OK, so we've calculated all of those values, those annual values of these various quantities that we selected here. Now we're going to extract them, and they are now available to plot. You can see the period is 1958 to 2012. And the quantities we have are the atmospheric water vapor. We have the ocean surface temperature, ocean ice cover. We've got the planetary albedo. We've got surface air temperature. We've got sea surface temperature. And we can start plotting these and seeing what they look like.
Let's start out with the surface air temperature. So this is the global average surface air temperature for the model. And it should be plotting that up for us shortly. There it is. So we can expand that window a little bit. We can change the scale here. So let's go from 9 and 8.5 to 23 on the vertical scale, just to get a finer vertical scale on this.
So the red curve shows us how average global land temperatures are changing over time in the model. The blue curve shows us how the open ocean temperatures are changing the model. Open ocean is going to be warmer, as you can see, by several degrees than land. It's the part of the ocean surface that isn't frozen. And the ocean, in general, warms up more than the land and is warmer than land on average because it doesn't go to as high latitudes, particular in the southern hemisphere.
So ocean temperatures are going to be warmer than land temperatures. The open ocean, the blue curve, is warmer than the full ocean, which includes ice-covered regions of the ocean. It's the green curve. And the black is the global average temperature. It's the average of all the regions, whether they're open ocean, ice-covered ocean, or land. That's what the black curve represents. Let's zoom in on that curve.
So we can say we started out in 1958 with a global average temperature of about 13.8 degrees Celsius, roughly what the global average temperature is prior to the increase in CO2 that's taken place since then. And we've warmed up by 2010 or 2012 to a temperature that's close to 17.8 degrees. So we've gone from about 13.8 to about 17.8. We've warmed up by about 4 degrees Celsius in response to that instantaneous CO2 doubling that took place in 1958.
So you can see how long it's taking the global temperature to equilibrate to that increase in CO2-- many decades. Although we can see that we are asymptotically approaching a new equilibrium value. If we were to extend this several decades into the future, as it turns out, we would probably see a net warming of 4.5 degrees Celsius relative to that initial temperature of 13.8 degrees Celsius.
And that is consistent with the fact that this particular model-- the GISS climate model, the NASA Goddard Institute for Space Studies climate model from the 1980s-- has a relatively high climate sensitivity of about 4.5 degrees Celsius equilibrium climate sensitivity for CO2 doubling. And that's consistent with the transient result that we're seeing here, as the temperature is approaching its equilibrium response to that instantaneous CO2 doubling.
But we can look at many other quantities in this model, in addition to surface temperatures. And so that's what we'll do next.
Video: EdGCM Demo - part 6 (4:45)
PRESENTER: OK. So we looked at surface temperature. Let's look at some other quantities here. These are all global averages. We'll look at the global average snow cover over time.
And we can see that's expressed as percentage-- the percentage of the land surface area that's covered by snow. That's what it looks like. If we look at the global average, that's the black curve. We can zoom in on that. We go from 5 or so to 12.
The global snow cover is decreasing in percentage from about 11% in the annual mean to a little over 7%, as we might expect. As the Earth is warming, snow cover is decreasing. In fact, that's one of the complimentary observations that we looked at earlier in the course that in addition to the warming of the Earth, we see that global snow cover is decreasing. That's just as the models projected to as surface temperatures warm.
We can-- no, I won't save that image-- we could look at the ocean ice cover. And again, we'll focus in on the global mean, so let's try to focus on that black curve. We'll go from a minimum of 1 to a maximum of 5.
And that's the black curve is a global average ice cover, and it is decreasing significantly as the Earth is warming. Again, as we might expect and as we have seen in the observations. Global ice cover is decreasing over time fairly dramatically. Northern hemisphere snow cover, where we have widespread records, is decreasing significantly as the model projects it too. And of course surface temperatures are warming.
So we can look at various variables other than surface temperature in this model and get some sense of what else is going-- what else is going on in this model. What else does this model project that we could look to the observations and see if the things that the models project should happen as we increase CO2 concentrations are indeed happening.
And why don't we take a look at global mean precipitation. And global mean precipitation is increasing. It's wetter over the oceans than it is over land, and the global average, the average of land and ocean regions is the black curve.
If we zoom in on that curve, OK, that gives us an idea of how precipitation is changing. Precipitation, in this case, is expressed as millimeters per day. And in the global average, we've gone from a little over 3 millimeters of precipitation per day in 1958 to something approaching about 3 and 1/2 millimeters of precipitation per day in 2012.
So in the global average, precipitation is increasing. That is also one of the robust projections of climate models. As we increase the surface temperature of the Earth, warmer oceans evaporate more water vapor into the atmosphere. The rate of evaporation increases. And to conserve water, that means that the rate of precipitation has to increase as well. In other words, we get a more vigorous hydrological cycle-- faster evaporation and faster precipitation of water out of the atmosphere.
And so the models project that global precipitation should increase, but what we saw previously in the observations was that, in fact, precipitation is a variable where there are very large regional differences. Certain regions become wetter, but other regions become drier. And so this is a case where looking at the global average, looking at a single time series, is going to be somewhat misleading. We really need to go to the actual spatial patterns of response in the models to see if we can make sense of what the model is projecting, and so that's what we'll do next.
Video: EdGCM Demo - part 7 (2:49)
PRESENTER: OK, so we've now gone to the Maps option. Previously we looked at Time Series, which give us a single number averaged over some region of the globe, typically the entire globe or the oceans or the land regions. But we can also look at the detailed latitudinal and longitudinal structure of these trends in various variables produced from the model.
The easiest way to do that is to pick, say, a five-year period at the beginning of the simulation and a five-year period at the end of the simulation so that we average out some of the year-to-year fluctuations. And we have an early baseline that we can compare to some later average in the model.
And I've already computed averages for various variables here-- snow cover, precipitation, soil moisture, surface air temperature, ocean mixed layer temperature. I've computed those all for a base period of 1958 to 1963, the first five years of the model simulation. And now what I'm going to do is calculate the spatial patterns of those variables for the last five years of the simulation.
So let's do that right now-- 2008, '09, '10, '11, '12. We're going to calculate the averages over that period. And it's doing that right now. You can see it going through the individual months for each of those five years. And it's going to calculate the averages of all those variables for each of the grid boxes in this model.
And the model is fairly low resolution. So as we'll see, the individual latitude-longitude grid boxes are in the order of seven degrees latitude and longitude. So it's a pretty coarse description of the surface. State of the art climate models today are run at much higher resolutions. But this is an earlier climate model. And at that time, it was necessary to resolve the Earth's surface into fairly coarse latitude-longitude grid boxes to run the models efficiently.
So now we're going to take a look at the spatial patterns of some of these variables, and in particular, the difference between the most recent five years and the first five years of the simulation, giving us a sense of how the model simulation is projecting changes over time and over the surface of the Earth.
Video: EdGCM Demo - part 8 (2:46)
PRESENTER: OK. So I've extracted the last five years of the simulation, the period 2008 through 2012, the average for that five-year period. And I'm now going to read it into the Data Browser, where it's available to plot. Then as a baseline, I am going to take the first five years, the average over the first five years of the simulation, read that into the Data Browser.
And you can see that I've got each of these five variables now, Ocean Mixed-Layer Temperature, Precipitation, Snow Cover, Soil Moisture, and Surface Air Temperature, all annual averages for each of these two five-year periods. And what I'm going to do next is to take the difference.
Let me select Surface Air Temperature. We're going to take the difference between the last five years and the first five years of the simulation. And that'll give us the spatial pattern of the trend in temperature over the globe.
So I want Data one minus Data two. It's going to plot that out for me. Unfortunately, it uses a nonsensical color scale. So I am going to change the color scale so that it is more meaningful.
OK. So the warm colors all indicate warming. The cold colors would indicate cooling. Of course, the entire globe is warming in this simulation, warming more at high latitudes, particularly in the northern hemisphere, where, as we know, sea ice is decreasing markedly. And so that ice albedo feedback is kicking in, giving us that additional warming at high latitudes in the northern hemisphere.
And there's some interesting structure in the southern hemisphere, as well, perhaps a little bit more warming over the land regions than over most of the ocean regions, although the variations are fairly small. So that's the projected surface air temperature pattern.
Of course, as I've said before, this is a fairly primitive climate model by modern standards. And a lot of the more interesting variations in ocean circulation and atmospheric circulation that give us more complicated patterns of surface temperature changes in the projections of current state-of-the-art climate models are not really resolved in this relatively primitive model.
But that's what the surface pattern of warming looks like. And we can now look at the patterns of some other variables.
Video: EdGCM Demo - part 9 (4:55)
PRESENTER: OK. So now I've selected Precipitation. And we're going to look at the change in precipitation over the surface of the globe. "Annual" means surface-- precipitation. Differenced-- the first five years minus-- the last five years minus the first five years of the simulation.
OK-- Data one minus Data two. And this is what we get. Again, let's use a more sensible scale. So we'll go from minus 3.09 to plus 3.09.
So we can see that overall, precipitation is increasing over the globe, as we already saw when we plotted out the global average precipitation. But there are some strong regional variations. There is a concentration of increased precipitation in a band near the equator.
The largest increases in precipitation are near the equator, where we have the Intertropical Convergence Zone rising motion in the atmosphere. And since the surface is warmer and there's more water vapor in the atmosphere, we get more rainfall in the region where rainfall tends to occur, which is this Intertropical Convergence Zone.
But as we go to the subtropics, we can see large patches of white. And what that's indicating is that in fact, in the descending branch of the Hadley circulation, where we tend to see deserts, that region is actually expanding poleward somewhat. And so that region of drying is now expanding towards the poles. And that's why we see these large areas of at least small decreases in rainfall in subtropical regions, contrasting with the large increases we see closer to the equator.
And then as we get into higher latitudes again, you can start to see that there's a larger tendency, again, for increased precipitation in the subpolar latitudes. And that is associated with a migration poleward of the mid-latitude band of frontal precipitation in both hemispheres.
So when we look at the pattern of rainfall, there's a far richer pattern of regional variation that tells us that, in fact, if we want to understand projected changes in rainfall, it's important not to just look at global averages or hemispheric averages. But look at the underlying pattern of changes, which is fairly complex, even in this case.
But of course, this is a relatively simple model. In state-of-the-art models today, the patterns of projected change in variables like rainfall are even more complex, even more regionally variable, because these models are able to resolve important changes in ocean and atmosphere circulation that impact on regional precipitation, for example, changes in the El Niño-Southern Oscillation phenomenon, which has a large impact on regional patterns of rainfall.
But even in this fairly basic, this fairly primitive model from the 1980s, we can see this pattern of latitudinal variation in how rainfall changes. Even on the average, there is an increase in global rainfall. That change in rainfall is strongly regionally variable. And there are some regions in the subtropics where this model projects a modest decrease in rainfall.
So we'll leave our discussion of EdGCM there. This, again, is a relatively primitive climate model by modern standards. And yet we can see some of the changes that we know are projected by more state-of-the-art climate models, with regard to changes in temperature, changes in rainfall, changes in sea ice.
And so as we go on into our next couple of lessons and we start to look at projections of state-of-the-art climate models, we will see that many of these predictions with the earliest models are borne out by more realistic models that are available today.