
Energy vs. Environment?
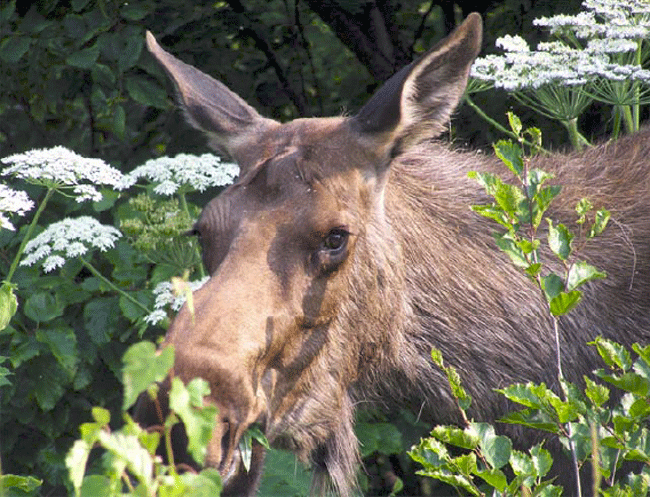
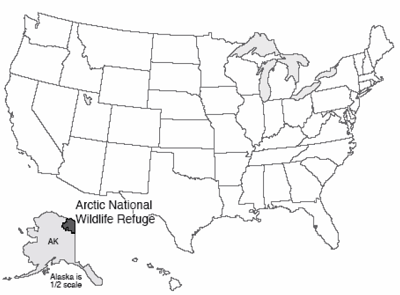
The Arctic National Wildlife Refuge (ANWR) sprawls across the North Slope of Alaska, from the Brooks Range to the coast of the Arctic Ocean, and is nearly as large as the state of Maine. ANWR is home to grizzly and polar bears, wolves loping across the tundra, moose and caribou, uncounted waterfowl, and snowy owls ghosting on white wings.
And beneath it, there probably is oil. The nearby Alaska Pipeline has pumped billions and billions of dollars worth of petroleum south from regions near the North Slope. But as that Prudhoe Bay oil runs out, the pipeline may soon be left empty--a very expensive conduit with nothing to carry. Similarity of geology suggests that ANWR also has oil to fill the pipeline, and to fuel automobiles in the U.S., or China, or somewhere. Not a lot of oil—maybe 10 billion barrels, according to the USGS. That is just over two years of U.S. oil imports at recent rates, not much more than one year of total U.S. oil use, and not exactly “energy independence,” but at $100 per barrel, it represents something like $1 trillion. The argument between wilderness and development has been going on for years, and is not ending soon; the recent emphasis on "fracking" for oil and gas in the lower-48 has temporarily shifted press attention away from the Arctic, industry interest in the north remains strong.
Fossil Fuels
Plants have an amazing ability. They take carbon dioxide (CO2) and water (H2O) and use energy from the sun to turn them into more plant material (which has an average chemical composition fairly similar to CH2O), releasing oxygen (O2) in the process. An approximate formula for photosynthesis is:
CO2+H2O+energy→CH2O+O2
Most of the rest of us—animals, fungi, many bacteria, as well as forest fires—run this reaction backwards, combining plant material with oxygen to release energy, carbon dioxide, and water. Done rapidly, this is “burning” in a fire; done slowly, it is still burning of a sort, which you might call "respiration." Plants usually include a bit of nitrogen that we didn't write in the simplified formula above, and animals often use the plant material with its nitrogen to make proteins that make animals, but after the animals die, they are almost always "burned" by bacteria or fungi or other animals that eat them to release the energy.
But, what happens if dead materials end up in a place without oxygen? It turns out that a lot of burning can be done by replacing oxygen with other things such as sulfate, but if these run out too, burning is no longer possible. Then, fossil fuels—coal, oil, and natural gas—become possible.
Coal is formed when bacteria break down dead plants. When there is no free oxygen in the air or water, bacteria remove the oxygen and hydrogen that are included in the plant material, leaving mostly carbon and forming a brown material called peat. When peat is buried by more sediment, heating and pressing drive off more and more of the little remaining oxygen and hydrogen, thus forming coal. Brown coal (lignite) has not been cooked much; it is common in the western United States. “Normal” or bituminous coal, formed from lignite by heat and pressure, is common in many places including western Pennsylvania. (This is a Penn State class, so we do keep track of Pennsylvania!) In a few places including eastern Pennsylvania, closer to the center of the old Appalachian Mountains, the bituminous coal was cooked to metamorphic anthracite coal. Peat is found with loose sediment, lignite with not-too-hard sedimentary rock, bituminous with harder sedimentary rock, and anthracite with metamorphic rock.
Oil is something like coal, but it is formed from dead algae buried in mud, usually from marine settings but sometimes from lakes. Algae start with more hydrogen and less oxygen than wood, so they produce a different fossil fuel. Heat breaks down the algae to release liquid oil. More heat breaks down the oil and makes natural gas, which is primarily methane (CH4). Some natural gas also is made at low temperatures by bacteria. While the heat is making oil and gas, the mud is being squeezed to make shale. Pennsylvania contains some oil and natural gas, and the first oil well in the world was drilled in western Pennsylvania. Humans had used petroleum before, but from natural seeps rather than wells. The push for petroleum that led to the first oil well was fueled by a looming shortage of whale oil, as the demand for that product far exceeded the ability of the oceans to grow the whales that produced the whale oil that was used to light homes on dark nights.
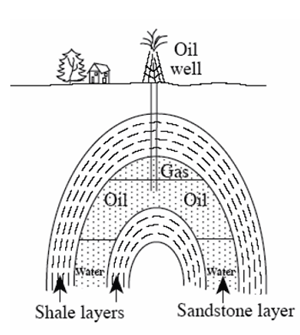
Oil and gas are low in density, float on water, and so tend to rise through water-filled pores in rocks and escape at natural seeps on land or beneath the sea. In some places such as off the U.S. Gulf of Mexico coast, biological communities have been found living on oil seeps on the sea floor. (Oil is natural, and nature uses oil in small quantities. But if a supertanker wrecks or an oil well blows out, nature cannot use all that oil at once, and problems result.) Because of the tendency for oil to escape, a large accumulation of oil can form only if there is a trap of some sort. Many different types of traps exist. For example, fluids do not pass through shale easily because it has only tiny spaces; if shale lying above sandstone is folded a little, oil and gas may be trapped in the sandstone layer, as shown in the figure.
At present rates of use, and at costs vaguely similar to what we see today, the oil and gas will last for most of a century, and the coal for a few centuries. If we were willing to pay more for gasoline, say $50 per gallon, more fossil fuels would be available. Although the store of fossil fuels is certainly limited, there is a rather large quantity. Until very recently, U.S. production had been dropping, causing us to import most of our oil from other countries, although as noted in the next section, "fracking" is affecting this. World production will probably peak in the next few decades (and some experts have suggested a peak within a few years). Demand for fossil fuels is rising rapidly, particularly in China and India as they industrialize. Shortages of fossil fuels, and worries about such shortages, already cause political problems; some observers believe that such worries are one reason the U.S. government spends so much on military preparedness.
Marcellus: Millions or Mayhem?
In Pennsylvania and some nearby states, there is much interest now in the natural gas being produced by "fracking" the Marcellus Shale, while Texas is fracking the Barnett Shale, North Dakota is fracking the Bakken Shale, and others are coming into play or being considered, in the U.S. and overseas. When a deep ocean ran out of oxygen back in the geologic past, mud and organic material accumulated on the sea floor, and these became black shales over time. Burial and heating broke down some of the organic material to make oil and gas, and some of that escaped from the shale, either leaking out at the sea floor or being trapped in special places that we have drilled into to get the fossil fuels.
But, a lot of organic material remains in the black shales, stuck between the very tiny clay particles that make up the shale, and not able to get out. People have learned how to drill into such layers, and then turn the drill to go along the layer. After a long hole is made, water and sand and various chemicals are pumped into the hole at very high pressure, breaking the rocks and then squirting into the new fractures to hold them open, in a process called “fracking.” Gas then leaks out along the fractures and up the hole, where it is collected and used by people.
In the big picture, this doesn’t make a lot of difference—the estimates of the total amount of fossil fuel that might be available have included the Marcellus gas since before the technology for recovering the gas became commercial, under the assumption that eventually we would figure out how to recover that gas. But, right now, there is much more gas on the market than there was a few years ago, and some oil is coming this way, some people are getting jobs or checks from gas companies for access to the land, other people are worried about the effects of the gas on the environment, and there is a lot of heated discussion going on. If you want to learn more about this particular issue, see the Enrichment.
Cost-Benefit Analysis
Let’s be honest. We use fossil fuels for good reasons. Most of our energy is obtained from fossil fuels. We run washing machines, rather than hand-scrubbing our clothes, primarily with fossil-fuel energy. Most of us are freed from the manual labor of hoeing and shoveling to grow our food because fossil-fueled tractors plow and plant and cultivate and harvest. Many of us have been freed from freezing to death in the winter, or perishing of heat stroke in the summer, or dying because we can’t get to the hospital in an emergency, because of fossil fuels. Humans have so far largely avoided the Malthusian trap of having lots of kids who have lots of kids until we exceed our food supply and then starve, primarily through being clever and through using cheap energy. Our energy use in the U.S. is equivalent to each of us having more than 100 people working to take care of us.
For humans, and for the few types of domesticated animals that have benefited from our use of fossil fuels (pigs, rice, chickens and soybeans, for example), there is little question that fossil fuels have been good. For other species on Earth, our use of fossil fuels to tame much of the planet has been less beneficial. How much easier did trains make it for humans to travel west to shoot bison? How much easier is it to cut a tree with a chain saw than with a stone hatchet? However, this is complicated by the fact that we have let some trees grow back, and we quit burning whales (or whale oil) to light evenings because we switched to burning the long-dead algae and trees that are fossil fuel.
There clearly are other costs of fossil-fuel use. Marks of oil exploration on the tundra may last for decades or longer. Acid rain from coal-fired power plants has killed the trout in headwaters streams on the Laurel Ridge of central Pennsylvania, and in some other places. Smog is not good for us, and shortens our lives. However, our lifespan continues to lengthen, so the dangers of smog and other “modern” hazards, such as industrial chemicals and radiation, are less than the advantages of the technologies that gave us all of these things. In fact, our biggest dangers are probably infectious diseases (evolution of new nasties), each other (automobile accidents, murders, and wars) and ourselves (smoking and drinking and eating too much, while exercising too little) rather than the technological things or natural disasters so many people worry about.
The Greenhouse Effect
If not for the greenhouse effect, we humans probably would not be here. The Earth’s atmosphere allows the shortwave radiation (what we usually call "sunlight") coming from the sun to pass through to the Earth’s surface, without much interference. (There is a little interference. Among other things, blue light is scattered off air molecules a bit more than red light is, so the blue light bounces around in the atmosphere and reaches our eyes from all directions in the sky, whereas the red comes more directly from the sun, which is why the sky is blue.) The windows in a greenhouse similarly allow sunlight to enter easily.
But, the sunlight heats the Earth or the inside of a greenhouse, which then radiate longwave radiation back upwards. As we saw way back at the Redwoods, the total energy reaching the planet on average exactly equals the total energy leaving, but the arriving energy is mostly shortwave electromagnetic radiation (light that we can see) while the leaving energy is mostly longwave electromagnetic radiation (infrared radiation that we cannot see without special sensors). The windows of a greenhouse do not allow longwave radiation to pass through easily; some gets through, but some is trapped.
When the sun rises after a cold night, energy enters a greenhouse but has trouble leaving, and the extra energy warms the greenhouse. Warming makes the floor of the greenhouse emit more longwave radiation, forcing some through the glass until a new balance is reached between incoming and outgoing radiation, but with the greenhouse at a higher temperature than would occur without the windows of the greenhouse. Some gases in the atmosphere act in the same way as windows on the greenhouse, intercepting some of the outgoing longwave radiation and keeping that energy in the Earth system. Water vapor contributes the most to the greenhouse effect, but carbon dioxide, methane, and others also matter. Without these greenhouse gases in the atmosphere, the Earth would be mostly frozen. (For a brief meteorological perspective on greenhouses, see the Enrichment, which also explains why water vapor is a “slave” to the other greenhouse gases, so that carbon dioxide is more important for changing the climate.)
Human-Caused Greenhouse Warming
Human activities are increasing the concentrations of several greenhouse gases in the atmosphere. Carbon dioxide, from burning of fossil fuels, burning of forests, and a few other sources, is the greenhouse gas we hear the most about. Refrigerants (chlorofluorocarbons and related compounds) also are potent greenhouse gases, and are increasing in the atmosphere. Those refrigerants that are especially damaging to the ozone layer that protects us from harmful very-shortwave (ultraviolet) radiation are being phased out, but the less-ozone-damaging replacements are still greenhouse gases. Methane, produced in cow guts, landfills, rice paddies, and other places where carbon breaks down in the absence of abundant oxygen, also has been increasing. Eventually, the huge carbon-dioxide source and the long time that carbon dioxide survives in the atmosphere mean that carbon dioxide will dominate global warming.
The world is warming. Of this there is almost no question—thermometers, including thermometers far from cities and in weather balloons and on satellites, in the ground and in the ocean, analyzed by government and university scientists in many different countries, including with industrial funding, show that warming is occurring. So do the temperature-sensitive types of snow and ice (NOT the top of Antarctica at -40, which won't melt even with a fairly large warming, but we see reductions in the seasonal river and lake ice, seasonally and "permanently" frozen ground, springtime snow, mountain glaciers and more). The great majority of significant changes in where plants and animals live, and when during the year they do things, are in the direction expected from warming.
Furthermore, there is high confidence that the warming is from the carbon dioxide. The physics of warming from rising carbon dioxide is unavoidable. Must of this was actually worked out by the Air Force after World War II. They were not doing global warming, but instead were worrying about such things as the appropriate sensors on heat-seeking missiles to shoot down enemy bombers. Use the wrong sensor, and you can't "see" the target because carbon dioxide is in the way. And carbon dioxide interacts with infrared radiation going from Earth to space in the same way. Satellites confirm this every day. Scientists have worked very hard to find some other explanation of the warming, but if anything, the sun has dimmed a little over the last 30 years, while volcanic eruptions have thrown up particles that blocked the sun a bit, we have put up particles from smokestacks that help the volcanoes in blocking the sun and causing cooling, we have replaced dark forest with more-reflective crops to cause a little cooling, and nature has not done anything with cosmic rays or space dust or anything else that would explain what is happening. Indeed, we see the warming from rising greenhouse gases despite the other influences primarily pushing towards cooling; really, the best answer to "How much of the warming did human-released greenhouse-gases cause?" is "More than all of it, because other things have reduced the warming." Finally, the "fingerprint" of the warming (for example, warming down here but cooling high in the stratosphere) is just what is expected from the effects of greenhouse gases, and completely unlike the pattern expected from changes in the sun, volcanoes, El Niño, or other natural fluctuations. Computer models of the climate system, when forced with the known natural causes of climate change such as changes in the sun and volcanoes, do a pretty good job of simulating the climate changes that happened before greenhouse gases had risen much but do a lousy job of simulating recent changes; adding greenhouse-gas effects causes the models to simulate what happened quite accurately.
Even prominent “skeptics” have now publicly admitted the high probability that humans are warming the world. (One of the well-known, often-seen-on-Fox-News skeptics directly stated this during a public debate with Dr. Alley a few years ago.) If you follow the news, you know that there is a lot of argument about how large the warming will be and whether it will be good or bad, although scientific consensus is very strong that the warming will be large compared to recent natural changes, and will be more bad than good for humans and other living things.
Some of the argument involves feedbacks. Feedbacks are “extra” processes in a “system” such as the Earth’s climate. If you force a system to change by doing something to it, many other things may then change. Some of these will amplify what you just did, making the changes bigger than you could have accomplished by yourself; these are positive feedbacks. Others will oppose what you just did, making the changes smaller than what you initially forced; these are negative feedbacks.
You, for example, have all sorts of negative feedbacks built in. If you are placed in a warmer room (the forcing), your body will begin to warm up. But then a negative feedback kicks in—you start to sweat, and that cools you off. Your body temperature doesn’t change nearly as much as the temperature outside of you changed. But, if you have certain diseases, they may fool your body so that its negative feedbacks are reduced and may even become positive feedbacks. Fever is usually a good thing, helping the body fight invading germs more effectively, but people die of fever when the feedbacks become positive and the body “burns itself up.” If you’re in a canoe with a really enthusiastic golden retriever, you may try to lean as the dog leaps about in such a way as to stabilize the canoe—you are providing a negative feedback on the tipping. But if the dog tips the canoe, and the ice chest falls to the low side, the ice chest is acting as a positive feedback to amplify the dog’s motion and tip the canoe further. If you lose your balance when the dog lunges to the side, you may suddenly fall toward the dog, providing another positive feedback and perhaps flipping the canoe.
The Earth certainly has positive and negative feedbacks. The easiest stabilizing one is the very fast change in radiation—a warmer place glows more brightly and sends more heat toward space, tending to cool the hotter places faster. Other than this almost-instantaneous change, most of the feedbacks over times that matter to us (years through millennia) are positive, amplifying changes; over still-longer times (say, millions of years), the feedbacks tend to be negative, stabilizing the climate. Climate changes over years or centuries thus can be almost as large as climate changes over millions or billions of years.
Put some extra carbon dioxide in the air and the climate will become warmer, speeding the rock-weathering chemical reactions that remove the carbon dioxide to produce dissolved ions that are used to make shells, removing the extra carbon dioxide. This negative feedback, which was explained by Penn State’s famous professor Jim Kasting and coworkers, stabilizes the Earth’s climate. Given too much atmospheric carbon dioxide, the excess is removed through warming-enhanced weathering; if too little, low temperatures slow weathering and allow carbon dioxide released by volcanoes from subducted shells to build up in the atmosphere. However, this takes hundreds of thousands of years or longer to act; over mere centuries, increased weathering will have little effect on the carbon dioxide we release, much of which will stay up for centuries, millennia or longer.
If we put carbon dioxide into the air and warm the Earth a little, several positive feedbacks begin to function. Warmer air can “hold” more water vapor (the saturation vapor pressure roughly doubles for a 10oC or 18oF warming), and water vapor is an important greenhouse gas, so warming causes more warming. Some of the shortwave radiation from the sun that hits the Earth bounces right back to space without first warming the Earth. This occurs especially over snow and ice, which have very high albedo or reflectivity. But, warming the Earth removes some snow and ice, which then allows more of the shortwave radiation to be absorbed, which warms the Earth more—a positive feedback.
Clouds reflect some sunlight (so cloudy days are cool), but clouds also interfere with outgoing longwave radiation (so cloudy nights are warm). The largest uncertainties in predicting how much warming will result from a given amount of fossil-fuel burning are probably related to how clouds will change, and whether these changes will produce positive or negative feedbacks. However, these uncertainties are not nearly large enough to affect the conclusion that future warming from fossil-fuel burning is highly likely.
We clearly wish to predict the future. If burning of fossil fuels, combined with bovine belches and leaky refrigerators, are going to cause too many problems, we might want to change our ways now. To predict the future, we need to do experiments. But, we have only one world. We cannot look at many different futures of one world, nor do we wish to wait many decades for the experiments to end. The solution we use is to build little worlds in computers, and run the experiments on those.
Geologists are important in this effort in two ways: we help find out how the world works, so that the right things can be put in the computers. The computer models always will be simpler than the real world, so careful choices must be made about what to put in. And, we provide data against which the models can be tested. You wouldn’t trust a model that had never been tested, but you wouldn’t want to wait a whole lifetime for a test. If the models can successfully simulate very different, warmer and colder climates of the past, then the models are probably pretty good. So we need to know about climates of the past.
The computer models of today actually are doing very well at “retrodicting” climate, predicting things that already happened. Modelers set up the configuration of ice sheets and ocean and continental positions and orbits and solar brightness, then model the climate and see if the computer results can match the climate that is recorded by the fossils and other climatic indicators in the rock record without “cheating” (so you can’t go in and tweak a lot of things to make the model match the data and then claim that the model is great—the models actually do work on past climates without such cheating). The models are also doing quite well at predicting the patterns of change we have observed with instruments over the last decades. Predictions made by modelers over the last decades are really occurring now. (For a little more on this, see the Enrichment, which also gives a bit more on why each doubling of CO2 has about the same effect on temperature, as covered next.)
Models predict that the world will warm about 3oC or just over 5oF for a doubling of CO2. The full warming will be delayed a few decades behind the rise in CO2, because the air can’t warm all the way until the ocean and ground have warmed and some ice has melted, which takes a while. The global warming to date, somewhere between 1oF and 1oC, is similar to the typical temperature variability at most places, so you really have to be paying attention to know that anything is changing. If we proceed to burn all the fossil fuels, something like an 8-fold increase above “natural” levels is possible over the next few centuries, or a warming of about 9oC or over 16oF, large enough that no one would have any doubt about the change. As noted in the Enrichment, uncertainty in the models is about 50%—the models project that the warming may be only about half this much, or more than one-and-a-half times this much, if we continue with business as usual and octuple CO2. However, because data on past climates are very difficult to explain if the models showing smaller changes are correct but are easy to explain if the models showing mid-range or large changes are correct, the low-end changes are less likely than the high-end changes.
So What?
Few people care about the temperature, and many care about what the temperature means for humans and other living things. Impacts are harder to estimate, both because of the additional uncertainties involved in turning temperature change into economic change, and because of the involvement of human values. For example, if warming causes species to become extinct, but those species were not economically important, how bad is that? You will find really strong differences of opinion across the political spectrum in the U.S. and the world.
Some disagreement is expected. Many places are already too hot for comfortable human habitation; making them hotter will not be good. Other places are too cold for comfortable human habitation today, so warming them might be good for humans there, unless you melt their ice and flood the coasts. Warming may remove one of the barriers that help keep malaria and other tropical diseases from spreading into today’s temperate zones, which is not good. Sea-level rise from warming is almost inevitable, as glaciers melt and the warming causes the ocean water to expand. Drying of the great grain-growing regions of the world during summers is likely. As a crude summary, most models indicate that human-induced warming will make our lives harder. People in the developed world are likely to cope; some in the developing world will cope, and others may fail (and die). A little warming is likely to hurt most of the world’s people (who live in too-warm places) but help most of the world’s economy (which is concentrated in fewer people in colder climates); too much warming then hurts everyone. A lot of loud radio commentators focus on the slight possibility of good outcomes, but ignore the possibility of disaster. (For a bit more on this, see the Enrichment.) Many professionals in the field note the benefits of caution—slow the ship in the fog until you have time to see how many icebergs are ahead, while starting to turn now to avoid the icebergs we already see.
Much of the argument focuses on economic concepts such as discount rates. Economically, something in the future is worth less than something today because of uncertainty (an apple in 30 years may do me little good, because I may be dead). If one uses this sort of reasoning, then any environmental change that takes more than a few decades to occur is of little concern—we humans will just deal with it when the time comes, using the great economic engine we’ve built.
But some people argue that intergenerational transfers should be treated differently—“we” humans who will “deal with” the changes will be our grandchildren, not us. Do we have the right to give them a greatly altered (probably degraded) Earth? Thus far, classical economic reasoning is “winning” in the real world, but the best science says that humanity will be better off if the reality of climate change is included in the decision-making.
Serious scholarship shows that, with a few decades of real investment in science and engineering, abundant energy can be provided while controlling greenhouse gases for a cost of roughly 1% of the world economy. That is in line with the costs of other clean-ups—sewers and garbage and catalytic converters and others. That is much smaller than most recent estimates of the coming damages from global warming if we do nothing. That also is a whole lot of money—roughly $400 billion per year now. Depending on one’s politics, the clean-up of greenhouse gases can be presented as a money-making prospect, as a low-cost obligation to the future, or as frighteningly expensive. Eventually, the switch away from fossil fuels must be made, because the fossil fuels are finite. And one can argue that $400 billion per year is a potential future industry that merits a real investment in research now. If we move away from fossil fuels, good jobs will be lost in fossil-fuel industries, but most studies point to a gain of more jobs in other fields. Note the problem, though—people in the industry who are worried about losing their jobs know who they are, and vote. People who will get the new jobs are students now, and don't know about the new jobs. So, there is some learning to be done.