Prioritize...
After completing this page, you should be able to discuss the controllers of evaporation rates and condensation rates. You should also be able to define and discuss relative humidity in terms of evaporation rates and condensation rates, and discuss the state of equilibrium.
Read...
In the last section, I asserted something that may have been surprising: evaporation and condensation are occurring around you simultaneously all the time, but you often don't see the results because they're happening on the molecular level. Obvious phase changes occur when there's either "net" condensation or "net" evaporation (assuming you have some liquid water to begin with). "Net" condensation means that the condensation rate exceeds the evaporation rate causing liquid water droplets to form. On the other hand, assuming you have some liquid water present to begin with, "net" evaporation, which means that the evaporation rate exceeds the condensation rate, causes liquid water droplets to shrink (or disappear altogether), or puddles on the ground to dry up, etc.
The states of net evaporation and net condensation are extremely important to weather forecasters, because they have implications for cloud and precipitation formation, as well as evaporation of precipitation (and subsequent evaporational cooling) among other things. To better understand how net evaporation and net condensation are achieved, we need to understand a bit more about what controls the evaporation rate (the number of water molecules evaporating in a given area over a given time period) and the condensation rate (the number of water vapor molecules condensing into liquid water in a given area over a given time period).
For starters, the bonds that loosely connect water molecules in the liquid phase aren't all that strong, so occasionally, the natural vibration of water molecules breaks these bonds, resulting in evaporation. Of course, as you know, the vibration of molecules depends on temperature: the higher the temperature, the faster the molecular vibrations, and the more likely a liquid water molecule will break free from its neighbors and evaporate into water vapor. So, that means water temperature is a major controller of the evaporation rate. Lower water temperatures yield lower evaporation rates, while higher water temperature yield higher evaporation rates.
What about the condensation rate? To explore the controllers of the condensation rate, let's perform a little experiment, starting with a closed, empty container filled with dry air (no water vapor molecules). Now, let's pour some water into the container and see what happens. In time, the most energetic water molecules break the molecular bonds with their neighbors and evaporate into the space above the water, gradually increasing the number of water vapor molecules there. As time passes and as and more water molecules enter the vapor phase in the space above the water, some water vapor molecules condense back into liquid as they come in contact (by chance) with the interface between the liquid water and the air above.

Initially, the condensation rate is small because only few water vapor molecules are present, and the probability that any one of them will come in contact with the interface between air and water is low. In fact, the evaporation rate far exceeds the condensation rate early on (net evaporation occurs). But, as time goes on, and net evaporation continues, the air above the water contains an increasing number of water vapor molecules. As the number of water vapor molecules increases, the chance of a water vapor molecule contacting the interface between air and water and condensing back into liquid also increases, which translates to an increase in the condensation rate.
So, as the number of water vapor molecules increases in the air above the water, the condensation rate increases, too. The condensation rate will continue to increase until it matches the evaporation rate, which is a state called equilibrium, meaning the condensation rate equals the evaporation rate. At equilibrium, the temperature of the remaining water on the bottom of the container is lower than the temperature of the water that was present at the start of the experiment. That's because the most energetic water molecules evaporated, thereby lowering the average kinetic energy (in other words, the temperature) of the water left behind. Moreover, the temperature of the remaining water equals the temperature of the "air" above the water. This state of equilibrium, where the condensation rate equals the evaporation rate, is depicted on the left below.
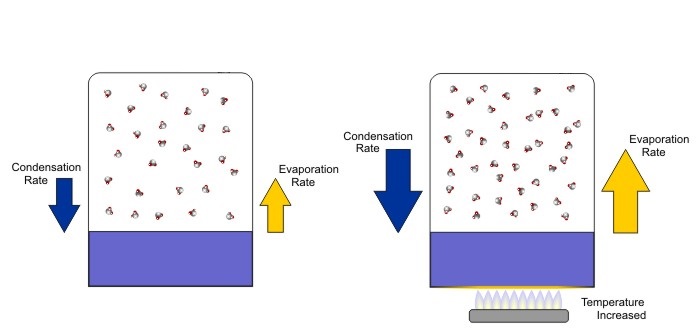
If we take our container in equilibrium and increase the temperature (depicted on the right above), what happens? The increase in water temperature causes the evaporation rate to increase and, for a time, net evaporation occurs. But, with increased evaporation, more water molecules exist in the air above the water, which in turn increases the condensation rate. The condensation rate again increases until it equals the evaporation rate, and a new equilibrium is achieved (with greater evaporation rates and condensation rates than the original equilibrium, shown above on the right).
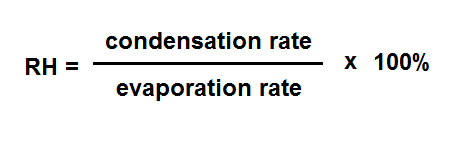
So, how do evaporation rates and condensation rates relate to weather? Well, they're the basis for a variable which perhaps you've heard of -- relative humidity. Although you may have heard the term "relative humidity" before, you may not know what it's really telling you. For starters, relative humidity is the rate of condensation divided by the rate of evaporation, multiplied by 100 percent (shown on the right). Relative humidity usually ranges from just a few percent (when the evaporation rate is much larger than the condensation rate) to 100 percent, which occurs at equilibrium. However, 100 percent is not the upper limit of relative humidity because, in reality, the condensation rate does sometimes exceed the evaporation rate slightly (that's how water droplets grow).
What does relative humidity tell us? It tells us how close the condensation rate is to the evaporation rate. As relative humidity nears 100 percent, the condensation rate nears the evaporation rate. Low relative humidity values mean that the evaporation rate greatly exceeds the condensation rate. But, because relative humidity depends on the evaporation rate, which depends on temperature, relative humidity doesn't tell us how much water vapor is present in the air. For example, the relative humidity is 100 percent in both stages of our experiment above in which the condensation rate equals the evaporation rate (equilibrium), but more water vapor molecules are present in the state of equilibrium after we've increased the temperature. By itself, relative humidity is also not a good indicator of how muggy or humid the air feels to most humans.
In practice, we can't calculate relative humidity using the equation above on the right because we can't easily determine the evaporation and condensation rates at any given time. However, we can relate evaporation and condensation rates to weather variables we can measure easily. Since we know that the condensation rate is controlled by the amount of water vapor present, and we use dew points to assess the amount of water vapor present, it stands to reason that condensation rates are connected to dew points. Indeed, higher dew points yield higher condensation rates. Meanwhile, temperature controls evaporation rates (higher temperatures yield higher evaporation rates), so relative humidity depends on dew point (which reflects the amount of water vapor present) and temperature. I should point out, however, that we can't just substitute dew point and temperature into the equation for relative humidity above and do a simple calculation. The mathematical connections between condensation rates and dew point, and evaporation rates and temperature are too complex for that, and are beyond the scope of this course. Still, understanding the basic connections between temperature and evaporation rates, and dew point and condensation rates leads us to the following important lesson learned:
This lesson has many important applications. For starters, it helps us understand the assertion I made in the last section that the potential for evaporational cooling when rain falls is greatest when there's a large difference between temperature and dew point. When there's a large difference between temperature and dew point, the rate of evaporation is much larger than the rate of condensation (relative humidity is low), meaning there's large net evaporation, which causes notable cooling.
These concepts also help us understand the conditions needed for cloud formation (net condensation). We'll be exploring how net condensation is achieved for cloud formation in the coming sections, but commonly, you may hear some folks erroneously explain cloud formation by saying that "clouds form as air cools because cold air can't hold as much water vapor as warm air." But, that's not really true -- it all comes down to evaporation and condensation rates. In the next section, we're going to explore the fallacy of warm air holding more water vapor than cold air.