Prioritize...
When you've finished this page, you should be able to describe the processes of evaporation and condensation, as well as how/why these processes impact temperature. In particular, you should be able to explain temperature and dew point changes that often occur with falling precipitation.
Read...
Since evaporation and condensation are such important phase changes for water, they deserve more of our attention. I briefly defined them in the previous section, but now I want to take a closer look at how these processes actually work, and what their consequences are for some weather variables that we've already talked about -- temperature and dew point.
For starters, evaporation is the process by which liquid water molecules break the bonds with neighboring molecules and escape into the air as water vapor, and as I mentioned briefly in the last lesson, evaporation is a cooling process, for a couple of reasons. First, water molecules with the greatest kinetic energy (fastest vibrations) are most likely break the bonds with their neighbors and evaporate, which means the average kinetic energy of the remaining liquid water is reduced (because the most energetic molecules are no longer liquid). A lower kinetic energy of the remaining water means a lower water temperature. Secondly, the breaking of bonds between liquid water molecules requires energy, and that energy comes from the surrounding air.
All of water's phase changes actually either use energy from the surrounding air, or release energy to the surrounding air, as illustrated by the "energy staircase" diagram for ice, water, and water vapor below. Although the diagram includes all of water's possible phase changes, we're going to focus on the two of greatest interest to us for now -- evaporation and condensation. If we start with liquid water, a few highly energetic, free-spirited water molecules can eventually break the bonds with surrounding molecules over time and escape to the vapor phase. Energy is required (600 calories per gram, to be exact) to break all the bonds to allow all the water to rather quickly evaporate and enter the gaseous phase of water vapor (the highest energy step), which cools the surrounding air.
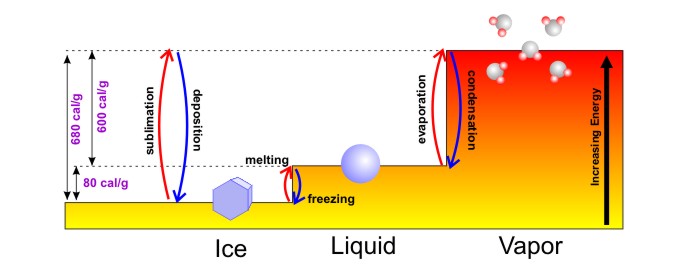
So, if evaporation is a cooling process, what about its reverse -- condensation (the process by which water vapor changes to liquid)? When water vapor condenses back into water, there's a step down in energy levels, so if you're thinking that condensation is a warming process, you're correct! Indeed, the energy used to evaporate water in the first place is never lost (a consequence of the conservation of energy), so as water vapor condenses into liquid water and bonds form between molecules, energy is released (600 calories per gram -- identical to the amount required for evaporation) to keep the energy books balanced. The release of this energy, called "latent heat of condensation," warms up the surrounding air.
So, any time a phase change (such as evaporation) causes water to go "up the energy staircase," energy is required to break bonds between molecules, which cools the surrounding air. Any time a phase change (such as condensation) causes water to go "down the energy staircase," energy is released, which warms up the surrounding air.
The warming that occurs with condensation is not easily noticeable to humans, but I bet you've noticed the impacts of evaporational cooling. When you get out of a swimming pool on a hot day, water drops on your skin begin to evaporate, which cools your skin. You've also noticed evaporational cooling in action if you've ever felt a rush of cool air before a shower or thunderstorm arrives. Indeed, temperatures often decrease just before, and after rain arrives. That's because the smallest raindrops evaporate along their descent to the ground, which extracts energy from the surrounding air.
To see a real-life example, check out the graph below, which plots surface temperatures and dew points at Louisville, Kentucky on June 11, 2014. I've highlighted a sharp drop in temperature (black line) that occurred in the middle of the afternoon, between 1500 and 1600 local time (between 3 P.M. and 4 P.M.). At 3 P.M. (15:00 on the graph), Louisville reported a temperature of 81 degrees Fahrenheit, but an hour later, the temperature was only 73 degrees Fahrenheit.
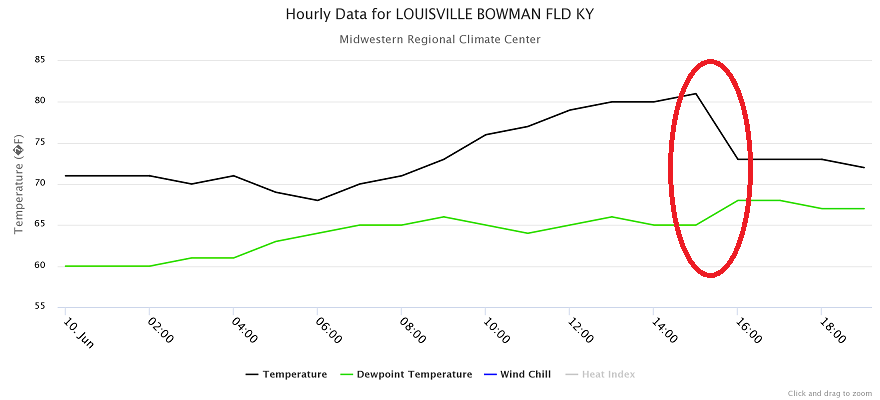
Why did the temperature fall during this hour? Evaporational cooling! It started to rain between 3 P.M. and 4 P.M., and during that hour, 0.13 inches of rain fell in Louisville, but as precipitation began, evaporation of rain drops cooled the air and temperatures decreased. Also note that dew points increased during the time highlighted in the graph above. Why is that? Well, if liquid raindrops were evaporating into water vapor, that means more water vapor was present in the air, and as you may recall, higher concentrations of water vapor go along with higher dew points.
You probably don't realize it, but evaporation and condensation are occurring around you simultaneously all the time! You just can't see the results because they're happening on the molecular level. Obvious phase changes occur when there's "net" condensation, meaning that the condensation rate exceeds the evaporation rate (liquid water droplets form), or if there's "net" evaporation (assuming you have some liquid water to start with), which means that the evaporation rate exceeds the condensation rate. The evaporation of rain drops on their descent to the ground is a great example of net evaporation. Tiny raindrops end up shrinking or disappearing altogether as the rate of evaporation exceeds the rate of condensation.
I should point out that the potential for evaporational cooling is greatest when a large difference between temperature and dew point exists because large differences between temperature and dew point allow for the greatest net evaporation. As temperatures and dew points get closer, net evaporation is reduced, which yields less evaporational cooling. To really understand why this is the case, we need to explore what controls the rates of evaporation and condensation. We'll do that in the next section, as well as see why comparing evaporation rates and condensation rates is so important to weather forecasters. Read on!