
What Glaciers Do, Erosion and Yosemite
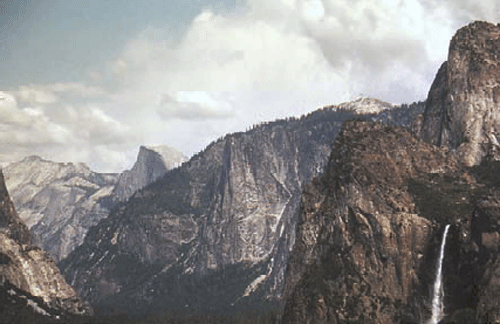
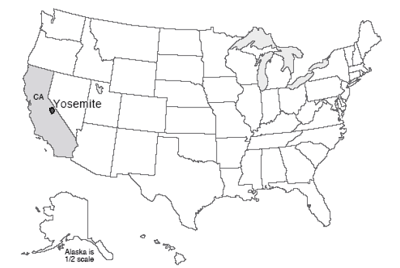
When your tour guide, Dr. Alley, was a much younger man (the year he graduated from high school, 1976), he traveled with his sister Sharon and cousin Chuck on a camping tour of the great national parks of the American west (in Chuck’s 1962 Ford Galaxy 500 land boat). At Yosemite, they hiked from the valley up to Glacier Point. The trail switch-backs up the granite cliffs, opening increasingly spectacular panoramas across the great valley of the Merced River. The view from Glacier Point, across the side of Half Dome, and the thundering Vernal and Nevada Falls, is well worth the climb. It was here that John Muir helped convince President Theodore Roosevelt of the need for a National Park Service to care for the National Parks, which were protected by law but not by rangers for some decades after the parks were established.
The hikers were a bit disheartened by the crowd at Glacier Point—the view is also accessible by the Glacier Point Road. While they sat and lunched, a tour bus pulled in. Most of the passengers headed for the gift shop, but three settled at a picnic table while a fourth strolled over to the railing to see the scenery for a few moments before joining the others at the picnic table. One of the quick-sitters asked “Anything out there?” To which the ‘energetic’ one replied “Nah, just a bunch of rocks. Let’s go check out the gift shop.” At last report, the gift shop had been removed. Regardless, it must be a sad person indeed who would not walk 50 feet to see the glory of Yosemite.
To anyone with open eyes, Yosemite Valley—the “Incomparable Valley”—is well worth inspection. It is carved from the granites and similar rocks of the high Sierra Nevada of California. Once, this granite was magma (melted rock below the surface), far beneath an earlier mountain range. The magma may have fed subduction-zone volcanoes much like those of the Cascades, which continue to the north of the Sierra. However, stratovolcanoes along this part of California have died as the East Pacific Rise spreading center ran into the trench along the west coast, forming the San Andreas Fault but ending subduction. Such a fate eventually awaits the Cascades volcanoes, some millions of years in the future.
The Sierra Nevada was raised and tilted along the great fault to its east, and looks down on Death Valley and the rest of the Great Basin. Earthquake activity, and faults cutting recent sediments, show that the mountain range is still being lifted above the still-dropping Great Basin.
The tough granite of the Sierra Nevada is more resistant to weathering and erosion than are most rocks, but granite does eventually break down, and some streams have managed to exploit weaknesses and cut deep channels through the range. These streams include the Tuolomne River, which carved the mighty Hetch Hetchy valley, now dammed so that a valley the equal of Yosemite is lost under water. The Merced River, which runs through Yosemite Valley, also cut into the range.
The stage was then set for the ice ages. Glaciers gathered on the high peaks, flowed into the valleys, and began to change the landscape.
Which Way Did It Flow?

A glacier is a mass of snow or ice that deforms and moves. Glaciers form wherever snowfall exceeds melting over enough years to make a pile big enough to flow. In places with extremely high snowfall, this can occur where average temperatures are near or even slightly above freezing, such as on the mountains of the Olympic Peninsula. In dry places, glaciers may be absent even if average temperatures are well below freezing. Such places have frozen ground instead, called permafrost (because the frost is permanent).
A pile of pancake batter spreads across a griddle, moving away from where the upper surface of the pile is highest. In the same way, a glacier moves from where its upper surface is highest to where its upper surface is lowest. In the figure below, the pressure at point A (the weight of the material above point A) is larger than at point B, because there is more ice above A than above B. The higher pressure at A gives a net push from A to B. Whether the diagram shows pancake batter, or the ice sheet on Antarctica, this push causes the material to deform and flow.
If you make a pile of pancake batter on a waffle iron, some of the batter may flow along the low grooves and then move up to cover the bumps, but the flow will always move away from the place where the upper surface of the pile is highest. In the same way, ice can flow up a hill in the bedrock if the flow is going in the “down” direction of the upper surface. For example, pieces of Canada are strewn across northeast Pennsylvania, and were brought across Lake Ontario and New York by the ice-age ice sheet. Ice at the bottom of that glacier climbed out of the low spot that now is the lake basin, driven by the upper surface of the ice sloping down from Canada to the U.S.
The total imbalance in pushes is larger for thicker ice than for thin. A very thin ice mass will not deform fast enough for the motion to be measured, and so is not considered to be a glacier. Typically, ice thicker than about 50 m (150 feet) will deform and flow, making a glacier.
Look at the second part of the figure. Glaciers move in one or more ways. All glaciers deform internally, like your slow pancake batter spreading on the griddle. A vertical hole drilled in a glacier will deform as shown in the figure. The stresses are largest, causing most-intense deformation (the permanent bending of the hole shown in the figure), in the deepest ice. The upper ice rides along on the deeper ice, so the velocity is fastest at the surface. Some glaciers are at the melting point at the bottom, warmed by heat flowing out of the Earth beneath. These melted-bed glaciers may move over the material beneath them, either by sliding over those materials (shown in the figure) or, if the materials are soft sediment, by deforming those sediments in a sort of slow landslide (not shown).
Recall that rivers adjust to move sediment and water from one place to another. So do glaciers. The water is supplied, frozen, in the accumulation zone, where snowfall exceeds melting, causing snow and ice to accumulate. The frozen water flows to the ablation zone, where melting exceeds snowfall (ablation means wearing away), or else flows to where icebergs break off (called calving) and drift away to melt elsewhere. For ice sheets covering continents or for smaller ice caps covering plateaus or mountain tops, the ice forms a dome and spreads out in all directions. For glaciers on the sides of mountains, the ice flows down the mountain—the upper and lower surfaces of the ice slope in the same direction.
When we talk about the advance and retreat of a glacier, we are referring to the position of its terminus, where the glacier ends by melting or calving. A glacier is advancing when it is getting longer, and retreating when it is getting shorter. Notice that ice almost always continues flowing from the accumulation zone to the terminus whether the glacier is advancing or retreating—retreat occurs when ice loss by melting or calving is faster than new ice is supplied, and advance occurs when ice is supplied more rapidly than it is removed by melting or calving.
Permanent deformation—flow—within ice may seem strange—after all, ice is a solid. But, as for the soft rock of the asthenosphere down in the mantle, or the soft chocolate bar in a hot pocket, or the red-hot horseshoe in the blacksmith’s shop, ice in all glaciers on Earth is nearly warm enough to melt, and so can flow slowly. As a general rule, materials heated more than halfway from absolute zero to their melting point can flow slowly, and flow becomes easier the closer the temperature is to the melting point. For ice, the coldest yearly average temperature on Earth is about eight-tenths of the way from absolute zero to the melting point, so ice at the Earth’s surface is “hot” and is able to flow. For more on this, and on the occurrence of crevasses as well as flow, see the Enrichment.
Glacier Tracks
A glacier frozen to the rock beneath does not erode much. However, thawed-bed glaciers, especially those with surface meltwater streams draining to their beds through holes (something like cave passages, although formed in different ways), can erode much more rapidly than streams or wind erode. Consider for a moment the Great Lakes of the U.S. and Canada. The lakes were carved by glaciers. The bedrock beneath Lakes Superior and Michigan is well below sea level, and was carved that deep by glaciers, not rivers! Today, rivers carry sediment into the Great Lakes, slowly filling them up. We will see later that over the last million years, times when glaciers were eroding have alternated with times when streams were filling the lakes back up with sediment, and the streams have had more filling-up time than the glaciers had eroding time. And yet, there are the lakes, not the least bit full of sediment. Evidently, the glaciers have been much better at their “job” than the streams have been. The same can be said for many other places. It is not too extreme to say that the regions glaciated 20,000 years ago and free of ice today still preserve a glacial landscape.
Ice moving over bedrock “plucks” rocks free, and then uses those rocks to abrade or “sandpaper” the bedrock, scratching and polishing it. As ice flows over a bedrock bump, the side the ice reaches first is abraded smooth while the other side is plucked rough. Subglacial streams sweep away the loose pieces, and may cut into the rock as well.
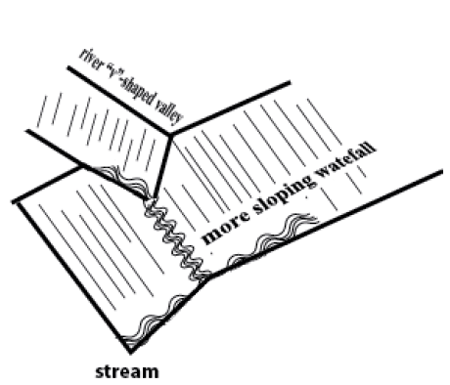
Plucked and abraded rocks show clearly that glaciers were present, but so do big features, as seen in Yosemite and elsewhere. If you could make a cut across a typical stream valley in the mountains, you would see that it usually is shaped like the letter “V”. The narrow stream cuts downward, and then mass-movement processes remove material from the walls, giving a “V”. (Where a V-shaped stream enters an ocean to make a delta, the outward and upward growth of the delta over time may eventually fill the bottom of the V with mud to make a flood plain, as we saw with the Mississippi, but initially, when a stream is cutting down, it makes a V.) However, glaciers are quite wide, and can erode across a broad region. Glaciated valleys exhibit a characteristic “U” shape. Yosemite Valley, with its near-vertical walls and near-horizontal floor, is a classic “U”, not a “V”.
The steeper a stream is, the faster it erodes. If a main river cuts down rapidly, then the side streams that flow into it will become very steep, and will cut downward very rapidly. In this way, even a small side stream can “keep up” with the main stream as it erodes downward, and stream processes usually produce “rapids,” rather than waterfalls where the side streams must plunge over cliffs to reach the main stream. Glaciers are different. A main glacier often fills its valley, the ice burying most or all of the rock. The ice from a side glacier then does not drop steeply down into the main glacier because there is no drop. So the side glacier is not steeper than the main glacier. The main glacier has more ice and rock and water than the side glacier, and so the main glacier erodes down more rapidly. When the ice melts, a “hanging valley” remains—a small stream that replaces the small side glacier must plunge over a glacially carved cliff and then flow across the bottom of the “U”-shaped valley to reach the main stream. Eventually, the side stream will wear away the waterfall. But today in Yosemite, numerous streams emerge from small “U”-shaped hanging valleys to cascade down the glacially carved cliffs—the landscape is pretty much what the glaciers left. (Piles of rocks at the bottoms of waterfalls show that the streams are indeed changing things, but slowly.)
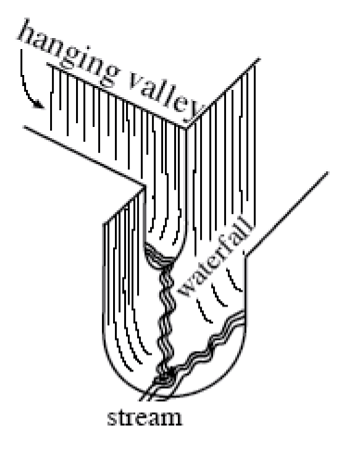
Glaciers make many other erosional features. At the head of a glacier, where freezing and thawing can break rock that is hauled away by the glacier, a bowl can be carved into the side of a mountain. If bowls chew into a mountain from opposite sides until they meet, a knife-edged ridge is left—the Garden Wall of the continental divide in Glacier National Park, which we’ll meet in the next chapter. Where three or more bowls intersect from different sides, a pinnacle of rock is left, such as the Matterhorn of Switzerland. Mountaineers have dubbed the bowls cirques, the ridges aretes, and the pillars horns, and geologists continue to use these terms.
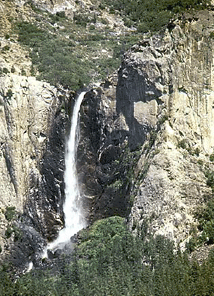
Glaciers also leave distinctive deposits. Streams, waves and wind all sort rocks by size, leaving too-big ones behind and carrying away smaller ones. Glaciers don’t care how big the rocks are that the ice carries, so a deposit put down directly from ice may have the tiniest clay particles mixed in with house-sized boulders. Such a deposit is called glacial till. Till plus glacial outwash (sediment washed out of a glacier by meltwater) may be piled up together in a ridge that outlines the glacier, called a moraine.
Pennsylvania has a Moraine State Park, which features glacial moraines. Cape Cod is a moraine, and a moraine is draped across Long Island, showing some of the places where glaciers from the ice age ended.