Prioritize...
At the completion of this section, you should be able to define and describe the eye and eye wall of a tropical cyclone (including how the eye forms). You should also be able to identify and explain the importance of the remaining three ingredients needed for tropical cyclones to form and thrive (weak vertical wind shear, a moist middle troposphere, and a neutrally stable or unstable troposphere).
Read...
In order to understand the importance of the remaining tropical-cyclone ingredients, we have to talk a bit about the "warm core" of these storms. As I mentioned previously, tropical cyclones are warm core systems -- tropospheric temperatures in and around the center of the storm are warmer than their surroundings (in contrast to mid-latitude cyclones, which are cold-core systems).
How does the warm core of a tropical cyclone develop? Organized thunderstorms around the center are the key. Simply put, the air parcels rising in thunderstorm updrafts are initially very warm and moist (due to evaporation from warm tropical seas). But, as these parcels rise and cool to form thunderstorm clouds, net condensation occurs, which releases energy to the surrounding air (called "latent heat of condensation"). So, air parcels cool as they rise, but the release of latent heat keeps them warmer than they otherwise would be, which keeps the air within a hurricane warmer than air at the same altitudes outside of the influence of the hurricane.
The release of latent heat is one important contributor to the warm core of a tropical cyclone, but it's not the only contributor. As air in thunderstorm updrafts reaches the top of the troposphere, it spreads out and flows outward from the center of the storm, creating divergence aloft, which as you may recall, reduces the weight of air columns near the center of the storm, and reduces the sea-level pressure. But, not all of the air near the top of the storm flows outward. Some drifts over the center and sinks. As the air sinks over the center of the storm, it warms (recall that the warming occurs as air parcels compress in environments of higher pressure as they sink). This sinking and warming air over the center of the storm contributes to the tropical cyclone's warm core, of course, but it also makes the tropical cyclone more intense because warmer air columns in the center of the storm are less dense, which further reduces sea-level pressure.
But, the consequences of sinking air over the center of a healthy tropical cyclone don't stop there! As air sinks and warms, relative humidity decreases as evaporation rates increase, which causes clouds to dissipate over the center of a healthy tropical cyclone. Indeed, sinking air over the center results in the formation of a tropical cyclone's "eye." For the record, the eye is a roughly circular, fair-weather zone at the center of a hurricane. By "fair weather", I mean that little or no precipitation occurs in the eye and an observer looking upward in the eye can often see some blue sky or stars. The visible satellite image of Hurricane Isabel from 1404Z on September 11, 2003 (below) shows a good example of a hurricane's eye. In general, a "healthier" hurricane has a very well-defined eye, and a deterioration of the eye (becoming obscured by high clouds) is often a sign of weakening.
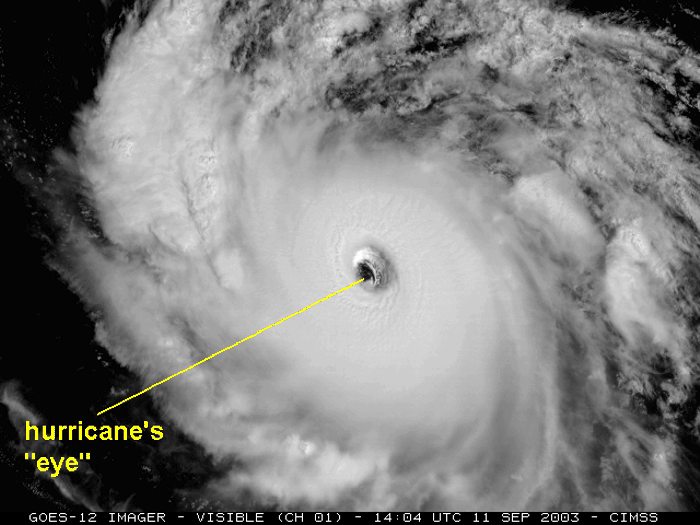
The diameter of the typical eye ranges from approximately 30 to 60 kilometers (about 16 to 32 nautical miles across), but eye diameters as small as four kilometers (approximately two nautical miles) and as large as 200 kilometers (approximately 110 nautical miles) have been observed. Immediately surrounding the eye is the eye wall, which is a ring of tall thunderstorms that typically contains the worst weather in a hurricane (most violent winds, etc.). So, the most violent weather in a hurricane typically surrounds the relative calm of the eye itself.
The combination of upper-level divergence and sinking, warming air over the center of tropical cyclones allows them to become the "kings of all low-pressure systems," attaining sea-level pressure values much lower than those of mid-latitude cyclones (assuming favorable environmental conditions, of course). For the record, Hurricane Wilma's "pinhole eye" was the smallest recollected by forecasters at the National Hurricane Center (two nautical miles) as the storm deepened to 882 millibars (the lowest on record in the Atlantic Basin) in 2005. For comparison, recall that most sea-level pressure values are greater than 950 millibars (even those in very strong mid-latitude cyclones). So, the organization of thunderstorms around the center is absolutely critical to the overall "health" of a tropical cyclone. With that in mind, let's explore the rest of our ingredients.
Weak Vertical Wind Shear
For starters, as a reminder, vertical wind shear is simply a change in wind direction and / or speed with increasing height. Weak vertical wind shear favors the development and maintenance of tropical cyclones, while strong vertical wind shear is detrimental. The reason that strong vertical wind shear (particularly wind shear directed in the opposite direction of the storm's motion) spells the kiss-of-death for tropical cyclones is that it disrupts convection around the center of the storm. In a worst-case scenario, large differences in wind direction and / or speed with increasing height can push the thunderstorms away from the center, exposing the low-level center of circulation, as happened with Tropical Storm Nicholas in 2003 (below).
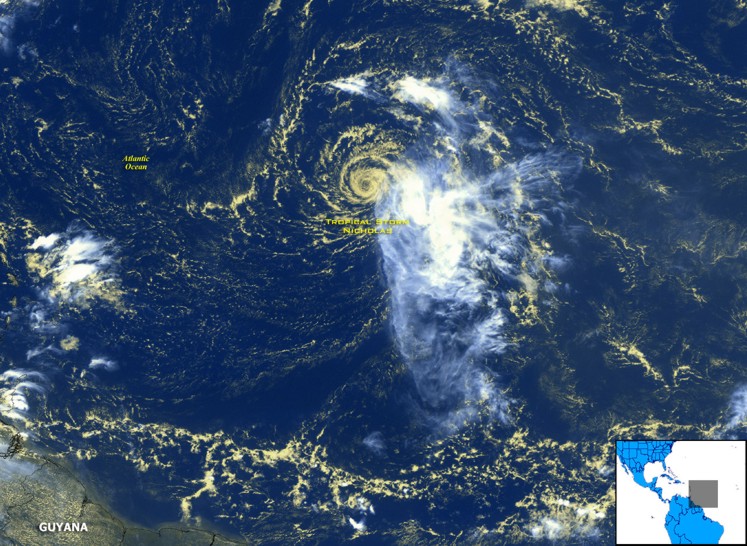
Note the swirl of yellowish low clouds that marks the low-level circulation of Tropical Storm Nicholas. Also note that the thunderstorms associated with Nicholas (bright white clouds) lie well to the southeast of the tropical storm's center. Given the lack of thunderstorms around the center of the storm, Nicholas was doomed and was downgraded to a tropical depression a few days later. Even when the impacts of shear aren't as dramatic as they were with Nicholas, at the very least the release of latent heat in thunderstorms and warming from nearby sinking air gets removed from the central region of the storm, which tends to increase surface pressure near the center of the storm and reduce the pressure gradient across the storm (which reduces the tropical cyclone's wind speeds).
For tropical cyclones, forecasters typically assess vertical wind shear in the layer between about 5,000 feet and 38,000 feet (often called "deep-layer shear" because the layer spans most of the depth of the troposphere). As a general rule, vertical wind shear values less than 10 meters per second (roughly 20 knots) between 5,000 feet and 38,000 feet are typically considered favorable for tropical cyclones to form and thrive. An important caveat regarding this threshold is in order, however. Like most meteorological thresholds, 10 meters per second isn't a magic value of wind shear at which the environment becomes unfavorable for all tropical cyclones. The impacts of wind shear depend on a few other factors, too, such as storm size. A small tropical cyclone, for example, may be "bothered" by shear even if it's a little less than 10 meters per second. A larger storm may not feel negative impacts from shear even if it's a bit higher than 10 meters per second. Think of 10 meters per second as a rough guideline, and not an absolutely firm threshold. The relative humidity in middle troposphere surrounding a storm can also help determine how resilient it is to vertical wind shear, which brings us to our next ingredient.
A Moist Middle Troposphere
Tropical cyclones tend to form and flourish in regions where there's high relative humidity in the middle troposphere. Conversely, middle tropospheric air with low relative humidity is like poison to tropical cyclones because of the process of dry entrainment, which you learned about previously. Recall that dry air gets drawn into cloudy air parcels during dry entrainment, which leads to net evaporation and cooling of the air parcel. Therefore, dry entrainment reduces positive buoyancy within thunderstorm clouds and encourages downdrafts, which stifles convection. Developing tropical cyclones are quite fragile, and when a developing disturbance entrains relatively dry air in the middle troposphere, it's unlikely to become a tropical cyclone. Indeed, mid-level dry air entrained into the central-core thunderstorms causes strong downdrafts to develop, which snuffs out new convection in the vicinity of the central core.
Strong, mature tropical cyclones have some limited resistance to environmental dry air because it "eats away" at thunderstorms on the periphery of the system at first, but once the drier air wraps into the storm's circulation, it deals a major blow to even strong tropical cyclones. Meteorologists sometimes use water vapor imagery to help them track regions of dry air in the middle and upper troposphere around tropical cyclones, and the two water vapor images below give an example. The image on the left shows Hurricane Isabel at 12Z on September 14, 2003. At the time, Isabel was a Category-5 storm, with maximum sustained winds of 135 knots. A little more than two days later (right image), Hurricane Isabel had weakened to a Category-2 storm (90 knots) after having ingested dry air in the middle troposphere. To watch the evolution, check out this loop of water-vapor images to get a better sense of how the dry air weakened Isabel. Note the dark splotch of relatively dry air over the Bahamas that erodes Isabel's western periphery and eventually wraps into the circulation of a noticeably weakening Isabel.
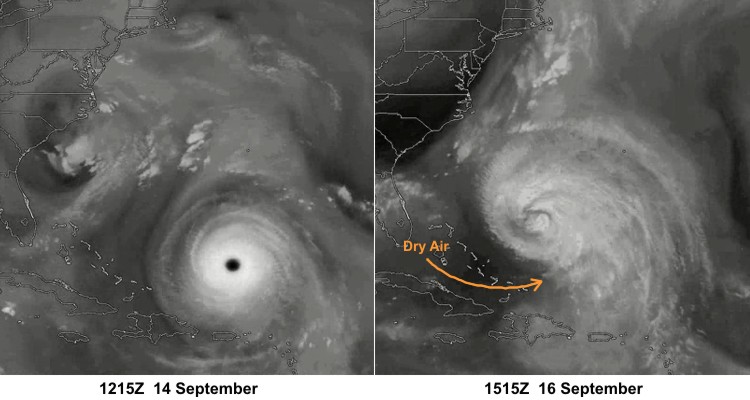
I should also point out that mid-tropospheric air with low relative humidity originating over land can also take its toll on a fully developed tropical cyclone as it approaches land. For example, on June 4, 2007, Super Cyclonic Storm Gonu in the Arabian Sea had maximum sustained winds that peaked at 140 knots. But, as dry mid-level air over the Middle East circulated into the storm, the storm fizzled to a Category 1 storm in a little more than 24 hours. So, ultimately, tropical cyclones will weaken if they draw in mid-tropospheric air with low relative humidity as they approach land (or are out over open water).
A Neutrally Stable or Unstable Troposphere
When tropical cyclones form, the background environment is usually neutrally stable or has some weak "conditional instability," meaning that "moist" air parcels (in which net condensation is occurring) are unstable. In other words, the environment must not be so stable that air parcels can't become positively buoyant (because that would inhibit thunderstorm development).
The presence of a neutrally stable or unstable atmosphere ties back somewhat to sea-surface temperatures. Over oceans where sea-surface temperatures are less than 26 degrees Celsius, the troposphere is typically too stable for thunderstorm development, but at higher sea-surface temperatures (26.5 degrees Celsius or more), a deep layer in the troposphere typically becomes neutrally stable or slightly unstable for moist air parcels. So, in addition to favoring high evaporation rates, high sea-surface temperatures also environmental favor lapse rates that are sufficient for tropical thunderstorms to develop and flourish.
As with many of the other ingredients, if sufficient lapse rates are lacking, thunderstorm development suffers (and so, too, does the tropical cyclone). Now that we've covered the ingredients needed for a tropical cyclone, let's see what happens when we mix all of the ingredients together to "cook up a storm." Read on!