Prioritize...
After completing this section, you should be able to describe the main force that creates the wind (the pressure-gradient force). You should also be able to identify the direction of the pressure gradient force given a map of isobars, and qualitatively relate the strength of the pressure gradient force to the speed of the wind.
Read...
The first step in analyzing the wind direction and speed at a given location is to identify all of the forces that play a part in moving the air. So, let's start with the most basic question: what force causes the air to move horizontally in the first place? In other words, what causes the wind to blow? As you might have guessed, since we've been discussing atmospheric pressure, the reason that air moves horizontally is related to pressure. Specifically, differences in pressure across the globe result in a force, called the "pressure gradient force" that sets air in motion. Let's explore.
The Pressure-Gradient Force
Recall that a low pressure system is a "lightweight" (the air column above the center of a low weighs less than any of the surrounding air columns) and a high pressure system is a "heavyweight" (the air column above the center of a high weighs more than any of the surrounding air columns). But, as we also learned, the sea-level pressure difference between a fairly strong high-pressure system and a strong low-pressure system usually isn't much more than about five percent. Still, it's this contrast in sea-level pressure (difference in column weights) between highs and lows that drives the wind.
To see what I mean, let's perform a simple experiment. A Plexiglas container (pictured below) has two compartments separated by a removable partition. There's more water in the left compartment than there is in the one on the right, translating to a greater weight of water on the left than on the right. Thus, there's higher water pressure on the bottom of the left compartment than on the bottom of the right compartment. If I remove the partition, there's a flow of water from higher pressure to lower pressure. In other words, the water, initially at rest while the partition was in place, accelerated from rest once I removed the partition.
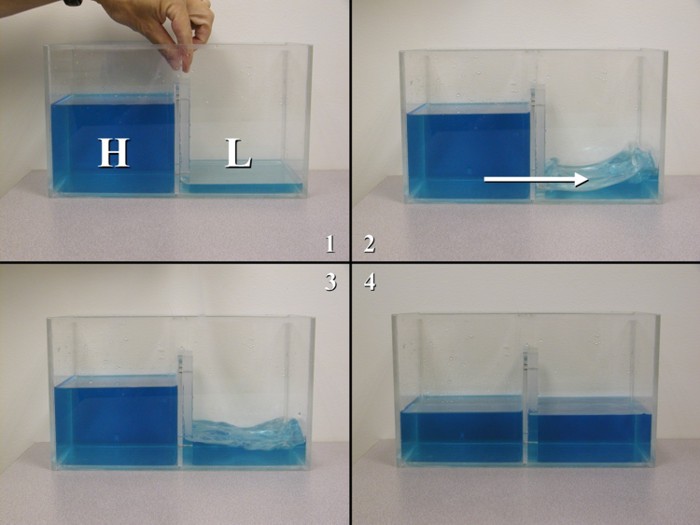
Isaac Newton's second law of motion states that when a net force is applied to an object, it accelerates; therefore, there must have been a net force acting on the water in order to set it into motion. In this case, the catalyst force was the pressure-gradient force, which acts from higher pressure toward lower pressure.
If the amounts of water in each compartment differ by a smaller amount, then the pressure-gradient force (PGF) is much smaller because the weights of the water in both compartments start out nearly the same. With a smaller pressure-gradient force, the flow of water will be much slower. Thus, we arrive at the following result: The magnitude of the pressure-gradient force (represented by the difference in water pressure across the partition in this experiment), dictates the speed of the flow of water.
In a sense, the atmosphere is like an "ocean of air," and switching our discussion from water to air gives the same result. Recall that the gradient of an atmospheric variable measures the difference over a given distance. So, if we're talking about the pressure gradient, we're measuring the difference in pressure over a certain distance. How do we assess the magnitude of the pressure gradient? You may recall that the pattern of isobars tells us how large or small the pressure gradient is:
- If isobars are packed very tightly (close together), that means there's a relatively large pressure change over a fixed distance, so the pressure gradient is large.
- If isobars are packed loosely (far apart), that means there's a relatively small pressure change over a fixed distance, so the pressure gradient is small.
I should note that, mathematically, the values of most sea-level pressure gradients seem small. Even tight packings of isobars (equating to a "strong" pressure gradient force) ultimately amount to a change of a tiny fraction of a millibar per mile. Yet, when isobars are tightly packed, wicked winds can blow! For example, check out the seal-level pressure gradient around the extremely strong 944-mb low-pressure system over the Bering Sea on November 9, 2011 (see 06Z surface analysis below). The pressure-gradient force caused winds to really whip, as you can tell from this YouTube video from Nome, Alaska (winds gusted over 50 miles per hour for several hours).
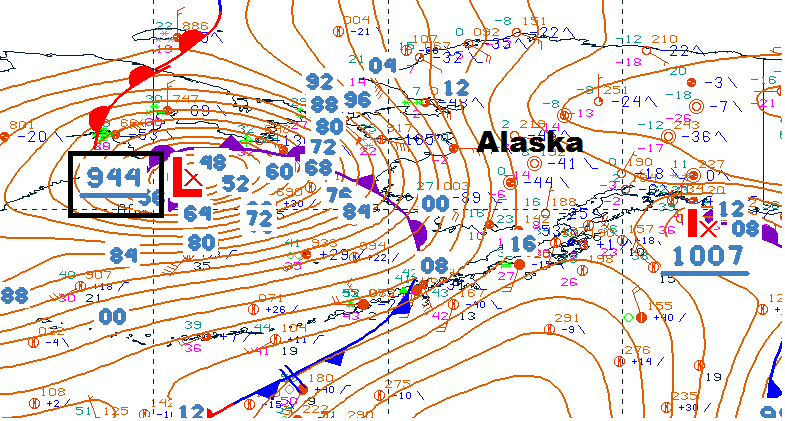
So, identifying areas with a relatively strong pressure-gradient force is as simple as finding areas on a map of sea-level pressure with where isobars are packed closely together. But, in what direction does the pressure-gradient force act? On maps of isobars, the pressure-gradient force is always directed perpendicularly to the isobars, and is directed from high to low pressure.
To see what I mean, check out the idealized surface weather map below. Ultimately, the pressure-gradient force has both a magnitude and a direction, so it's drawn as a vector that points from high to low pressure. Note how the orientation of the vector changes depending on the orientation of the isobars, but the pressure-gradient force always points perpendicular to local isobars, from higher toward lower pressure. The magnitude of the force is depicted by the length of the vector; note how the vector is longer where the isobars are packed closer together, indicating a stronger pressure-gradient force.
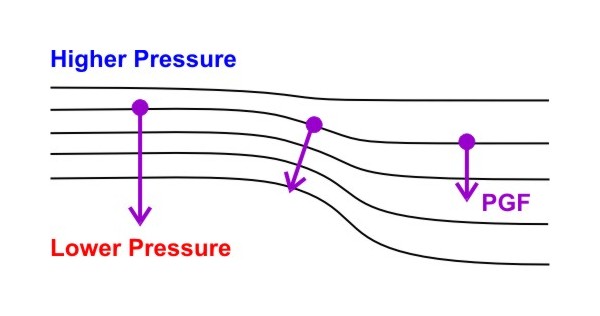
So, when isobars are packed closer together, the wind should blow faster, as the data from the intense low over the Bering Sea on November 9, 2011, indicate. The 12Z surface analysis from April 30, 2011 provides another good example. First, note the 40-knot sustained winds over eastern Montana and western North Dakota compared to the 10-15 knot sustained winds over eastern North Dakota (where the isobars are packed more loosely). You should also notice that the winds aren't blowing directly perpendicular to the isobars, from higher pressure to lower pressure. What's up with that?
The wind would blow directly from higher to lower pressure if the pressure-gradient force was the only force acting on the air, but that's not the case. We have to account for other forces as well when trying to assess wind direction and speed. We'll examine another one of those forces up next. Read on.