Prioritize...
After finishing this section, you should be able to define the term hydrostatic equilibrium (hydrostatic balance) and discuss the implications of hydrostatic equilibrium on vertical motions in the atmosphere. You should also be able to define buoyancy and the buoyancy force, as well as discuss under what conditions an object (like a parcel of air) is positively or negatively buoyant.
Read...
As you may recall, cooling is the most common way to make a cloud, and because air cools as it rises, upward motion in the atmosphere (rising air) is required for clouds and precipitation. When we studied mid-latitude cyclones, you saw a number of mechanisms that cause air to rise, such as overrunning and low-level convergence along a cold front. But, these mechanisms that cause rising air result in fairly slow upward motions, often roughly a few or several centimeters per second (at most maybe 10 to 20 centimeters per second). For reference, 10 centimeters per second is about 0.22 miles per hour.
To understand why vertical motions in the atmosphere tend to be quite gentle (even those that cause large areas of clouds and precipitation), we have to take a closer look at the vertical structure of the atmosphere. First, recall that pressure always decreases with increasing height. The vertical decrease in pressure naturally means that an upward pointing pressure-gradient force exists. In fact, the upward pointing pressure-gradient force is much stronger than the horizontal pressure-gradient force that drives the wind that we experience at the surface. Remember that even a large horizontal gradient in sea-level pressure amounts to a tiny fraction of a millibar per mile. But, the vertical pressure gradient is much, much larger. The pressure drops by more than 100 millibars just in the first mile above sea level!
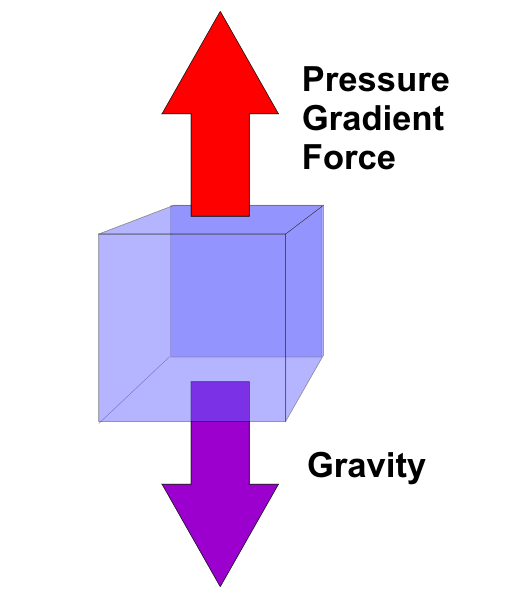
Given such a huge upward directed pressure-gradient force, why isn't there constantly a ferocious wind sucking everything upward off the face of the earth? The answer is that, on average, the downward force of gravity roughly balances the large vertical pressure-gradient force. This approximate balance between the vertical pressure-gradient force and gravity is called hydrostatic equilibrium (or hydrostatic balance).
Over large areas, the atmosphere exists approximately in hydrostatic equilibrium, which means that vertical accelerations tend to be small. With small vertical accelerations, upward and downward air motions also tend to be gentle (again, a few centimeters per second is common). But, not all vertical motions in the atmosphere are slow, especially those that occur over small areas. For example, the speed of rising air of cumulus clouds like the ones in this time-lapse movie over Mount Washington, New Hampshire are closer to 1 meter per second (a little over 2 miles per hour). That's still not super speedy, but it's much faster than a few centimeters per second!
Furthermore, updrafts in powerful thunderstorms can approach a whopping 50 meters per second (a little over 100 miles per hour). So, what's the mechanism for generating such fast speeds? Obviously, the atmosphere must deviate from hydrostatic equilibrium in order for air to accelerate upward to such impressive speeds, and we've already touched on the basic mechanism for generating such fast upward motions -- convection driven by buoyancy.
Recall that convection is the transfer of heat energy via the vertical movement of air, and to understand why air rises freely via convection, we have to review buoyancy. When an object is submerged in a fluid, the buoyancy force on the object is equal to the weight of the fluid displaced by the object. To put this in practical terms, suppose that, while taking a swim, you submerge your favorite beach ball and then let it go. What happens? Very quickly, the beach ball will bob to the surface of the water. In scientific terms, the beach ball is positively buoyant. Now submerge a rock and then release it. It falls to the bottom of the pool because the rock lacks sufficient positive buoyancy to keep it afloat. Formally, we say that the rock has negative buoyancy.
What makes the difference in the buoyancy between a rock and a beach ball? The answer is density. Formally, the density of an object is its mass (akin to weight) divided by its volume. The beach ball has a relatively large volume and small mass, making its density rather small and far less than the density of water. A rock, on the other hand, has a greater density than water, so it sinks. The magnitude of the buoyancy force depends on the difference in densities between the submersed object and the fluid - the greater the difference, the greater the buoyancy force.
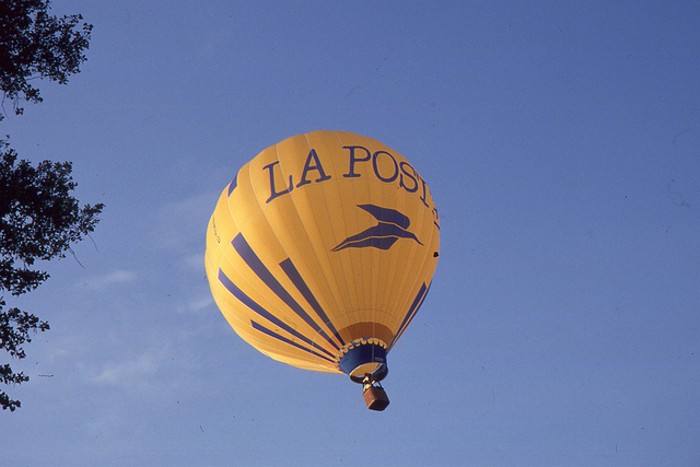
How then does buoyancy apply to the air? Well, when determining the buoyancy of the air, meteorologists often refer to "air parcels." I've used this term before, but as a reminder, a parcel is basically a small chunk of air (you could think of it as a bubble or a balloon filled with air). The air in the parcel initially has the same properties as the surrounding air; however, we'll assume that a parcel remains isolated from the surrounding atmosphere (except for pressure changes) when it moves vertically. That's not a perfectly realistic assumption, but it's very useful for meteorologists to draw conclusions about how the air will behave.
It turns out that the difference in temperature between an air parcel and its immediate environment governs the buoyancy of the parcel because the temperature and density of air parcels are related. A relatively warm parcel of air has a lower density than the cooler air surrounding it; therefore, it will be positively buoyant and have a tendency to rise. As the temperature (density) difference between an air parcel and its immediate environment increases, so does the buoyancy. So, relatively warm or hot air parcels are like beach balls that you drag to the bottom of a swimming pool. An inflated beach ball would be much less dense than the water at the bottom of a swimming pool, and therefore, positively buoyant. When you let go of the ball, it will race to the top of the pool.
By the same token, a relatively cool air parcel has a higher density than warmer air immediately surrounding it; therefore, it is negatively buoyant. In other words, it has a tendency to sink if it was initially at rest somewhere above the ground. So, cool air parcels are kind of like rocks that you might hold at the surface of a swimming pool. If you let go of the rock, the very dense rock (compared to the water in the pool) will sink to the bottom.
How high a positively buoyant parcel will rise (or how low a negatively buoyant parcel will sink from higher altitudes) depends, of course, on the density (temperature) of the parcel compared to the density (temperature) of its immediate, surrounding environment. Ultimately, a positively buoyant parcel will continue accelerating upward as long as it remains warmer than its immediate surroundings. But, you may recall that as air parcels rise, they expand and cool. So, parcels that accelerate upward to great heights and attain great upward speeds must end up cooling more slowly than their surrounding environments (so that the parcels remain warmer, by comparison).
Another way to think about this is that an environment in which temperatures decrease rapidly with increasing height helps air parcels remain positively buoyant longer because it becomes easier for a parcel to remain warmer than its surroundings as it rises. Such environments are often referred to by meteorologists as very "unstable." But, what does it really mean when a meteorologist says that the environment is "stable" or "unstable?" We'll explore that in the next section. Read on!