Prioritize...
By the end of this section, make sure that you can discuss how the earth's tilt on its axis of rotation impacts sun angle throughout the year, and how seasons result from the changing angle at which the sun strikes the earth. You should also be able to explain how "meteorological seasons" differ from astronomical seasons.
Read...
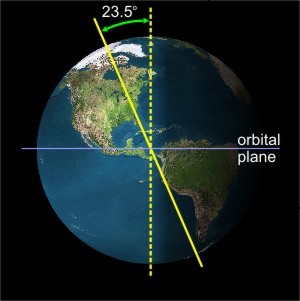
You've probably heard the saying, "It's a small world." But, that's not necessarily the case when we're talking about the seasons. If you live in the northern hemisphere, did you know that when it's winter where you are, it's summer in the southern hemisphere? It's as if the United States and Australia (for example) are worlds apart! While the depths of winter's chill numbs the middle latitudes of the northern hemisphere in January, temperatures can soar to the 90s during January in Australia (where it's summer). The reason for such seasonal disparity between hemispheres is that Earth's axis of rotation tilts at an angle of 23.5 degrees away from the line drawn perpendicular to the plane of our planet's orbit around the sun (as shown in the figure on the right).
The tilt of the earth's axis governs the heating power of the sun's energy. Just so you're not in the dark with regard to this claim, direct your attention to these side-by-side photos of a flashlight shining on a wall (credit: David Babb). Please note that when the light from the flashlight strikes the surface at a rather direct angle, the light focuses on a rather small area. In other words, the light is intense. On the other hand, if the flashlight is tilted so that the light strikes the surface at a lower angle, the light spreads over a larger area, making the light less intense. If you're not convinced, find a dark room and a flashlight and try it out for yourself.
The sun's radiation, for all practical purposes, travels in parallel "rays" of energy because of its distance from the Earth. Therefore, like our flashlight, the tilt of the earth controls the heating power of solar radiation and, on a grander scale, the seasons. So the tilt of Earth's axis of rotation explains the seasonal disparity between the northern and southern hemispheres, but it doesn't explain why we have four seasons (winter, spring, summer, and autumn).
To explain why we have a climate with four seasons, I'll start with the observation that the Earth's axis always points to the same position in space (namely, toward Polaris, the North Star). In addition, Earth travels in an elliptical orbit around the sun, which means that the northern hemisphere can be tilted toward or away from the sun, depending on its orbital position. To see what I mean, check out this interactive animation showing earth's orbit around the sun, and click on the "summer" text in the animation.
When the northern hemisphere tilts toward the sun, solar energy strikes the ground more directly during the daytime. Like a nearly downward pointing flashlight shining directly on the surface, concentrated sunlight has heating power consistent with the elevated temperatures of summer. Note that, at the start of summer, the Arctic Circle, which spans from about 66.5 degrees latitude to the North Pole, lies in daylight 24 hours a day. Thus, "nighttime" forecasts such as "partly sunny and cold" are not far-fetched in northern Alaska. For proof, check out this time-lapse movie of the Arctic sun and note that while it dips towards the horizon, it never sets!
At the same time the sun's rays are striking the ground more directly in the northern hemisphere, the southern hemisphere tilts away from the sun and sunlight strikes at a lower angle (like light from a nearly horizontally held flashlight spreading out over a large area). This diffuse sunlight has low heating power that is consistent with the typically low temperatures of winter.
Now click on "winter," "spring," and "fall" in the animation and investigate firsthand the effects of sun angle on seasonal heating power. I've also created a short video (3:45) using a virtual flashlight tool and the animation showing earth's orbit around the sun to discuss and summarize the reason for the seasons.
Click here for a transcript of the video.
Text on screen: The intensity of light that shines on a surface depends on the angle at which the beam strikes the surface. The shallower the angle, the more the light spreads out, resulting in a lower intensity. Grab the flashlight in the picture on the right and observe how the angle affects light intensity as you change the angle.
To understand the changing of the seasons, we have to understand the role of the changing Sun angle and the tilt of the Earth on its axis. To look at that, we're going to start with a virtual flashlight. We’ll begin with a situation where our virtual flashlight is pointed directly at an object, so the light comes in perpendicularly and strikes the object.
You can see that the light is fairly bright, and it's spread over a small area, so the amount of light per unit area is maximized, and the light is intense as it strikes the object. But if we change the angle of our flashlight and make the light come in at a lower angle and strike the surface, the light now gets spread out over a larger area. So the amount of light per unit area is reduced, and the light is dimmer on the surface. It's not as intense. So even though the same amount of light is coming out of the flashlight, it's dimmer on the surface because of the lower angle of the light that is striking the surface.
You could try that experiment at home with a real flashlight if you wanted. But in a nutshell, you've seen the reason for the change of seasons. Earth is tilted on its axis at a 23.5-degree angle, and as it revolves around the Sun, the location that gets the direct beam of light changes throughout the year.
The direct beam of light from the sun is always between 23.5 degrees north latitude and 23.5 degrees south latitude. For example, the equator gets the direct beam of light twice a year, on the spring equinox and on the autumnal equinox. On the other days of the year, the direct beam is over other various locations between 23.5 degrees north and 23.5 degrees south.
To better see how the changing Sun angle impacts the seasons, we have an animation here of the Earth revolving around the Sun, and the Earth is tilted on its axis at the 23.5-degree angle. The seasonal designations here are for the Northern Hemisphere. If we click on summer, we can see the situation in the Northern Hemisphere and why it's warmer in Northern Hemispheric summer.
When it's summer in the Northern Hemisphere, the Northern Hemisphere is tilted toward the Sun. So the Sun's light is striking the surface more directly and the heating power of the Sun is greater in the Northern Hemisphere during summer. Meanwhile, the Southern Hemisphere, it’s tilted away from the Sun, and the Sunlight is coming in at a lower angle and spreading out over a larger area. So, the heating power of the Sun is reduced in the Southern Hemisphere. That's why the Southern Hemisphere is having its winter while the Northern Hemisphere has its summer.
The situation during northern hemispheric winter is just the opposite. During the Northern Hemispheric winter, the Sun angle is low in the Northern Hemisphere, and the light is spreading out over a larger area, so the heating power of the Sun is reduced, because the Northern Hemisphere is tilted away from the Sun, and the light is coming in at a low angle.
Meanwhile, the Southern Hemisphere is pointed more directly at the Sun, so the sun is coming in more directly, and the heating power of the Sun is stronger in the Southern Hemisphere. That's why when it's winter in the Northern Hemisphere, it's summer and warmer in the Southern Hemisphere.
Finally, in spring and fall, the tilt of the earth's axis isn't directed toward or away from the sun, so the sun's rays strike similar latitudes at the same angle in both hemispheres, which means the radiation per unit area is the same and temperatures end up being similar. Ultimately, this set-up in spring and fall gives us an idea of what would happen if the earth was not tilted on its axis at all. We would not experience any seasonal variation as the earth revolves around the sun.
Astronomically speaking, the seasons break down like this for the northern hemisphere:
- Winter: Begins on the winter solstice (on or around December 22) when local midday rays of the sun shine at right angles on the Tropic of Capricorn (approximately 23.5 degrees South latitude).
- Spring: Begins when astronomical winter ends, on the spring equinox (on or around March 21) when local midday rays of the sun shine directly on the equator.
- Summer: Begins when astronomical spring ends, on the summer solstice (on or around June 22), when local midday rays of the sun shine directly on the Tropic of Cancer (approximately 23.5 degrees North latitude).
- Autumn: Begins when astronomical summer ends, on the autumnal equinox (on or around September 23), when local midday rays of the sun shine directly on the equator.

However, weather forecasters use different criteria to determine the "meteorological seasons" (also called "climatological seasons"). For example, meteorological winter in the northern hemisphere runs from December 1 to February 28 (or 29, if it's a leap year), a period that statistically includes the three coldest calendar months of the year. Meteorological summer in the northern hemisphere runs from June 1 to August 31, a period that includes the warmest three calendar months of the year. Meteorological spring and fall in the northern hemisphere (March 1 - May 31 and September 1 - November 30, respectively) mark the three-month periods that transition between the coldest and warmest seasons. So, meteorologists define their seasons by normal temperatures, not sun angle like astronomers do.
Of course, individual weather events can sometimes belie astronomical seasons (based on sun angle) and meteorological seasons (based on normal temperatures). To see what I mean, consider the average monthly snowfall for State College, PA. As expected, most of the snow occurs during meteorological winter (December, January, February). However, record snowstorms in State College tend to occur during the spring (not necessarily winter). Check out the graph to the left, showing the top-ten 2-day snowfall totals for State College. Five out of six of the largest snowstorms occurred in March, and the biggest storm on record occurred near the end of March,1942, when 30.5 inches crippled the region.
You should realize that while sun angle is the driving factor for seasonal temperature variations, there are other factors at play as well. For example, consider this NASA movie from 2000-2001, which shows that the rhythms of the most intense ultraviolet radiation coincide with the most direct rays of the sun (around the summer solstices). Of course, there's nothing surprising about this movie. But what may be surprising to you is that average air temperatures lag behind the astronomical lead of the sun's most direct days.
To see what I mean, let's look at the plot of annual average high temperatures for Pittsburgh, Pennsylvania (below). Note that the maximum daily temperature occurs in the latter half of July, on average, nearly a month after the summer solstice (the day when the midday rays of the sun strike Pittsburgh at their most direct angle). Similarly, the coldest days, on average, do not occur until the latter half of January, almost a month after the winter solstice. The bottom line here is that the greatest average daily high temperature at Pittsburgh does not occur on the day with the most intense radiation. Indeed, the greatest average daily high occurs about a month later when the sun's rays strike Pittsburgh at less direct angles.
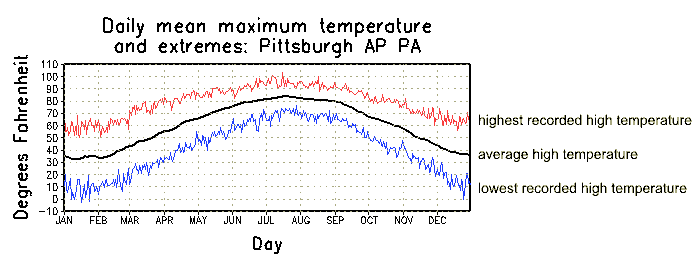
To understand why this happens in the most simple terms, imagine that you take a piece of cold pizza from the refrigerator and place it in a preheated oven. After a minute or so, you get impatient and remove the pizza from the oven. In salivating expectation, you take your first bite and are immediately annoyed that it's still cold. You turn up the oven to maximum and cook the pizza another minute, but it's still not piping hot.
Just as it takes time for cold pizza to heat up in a hot oven, the atmosphere, chilled by winter's refrigerator, takes time to warm up, not reaching its highest temperature, on average, until after incoming solar radiation reaches its maximum around the summer solstice. As we'll see a bit later, temperature lags often occur in the daily temperature cycle as well. That is, the maximum incoming solar radiation occurs near local noon but the high temperature for the day usually is recorded in the mid- to late-afternoon.
Now that you know the reason for the seasons, let's examine some other "big picture" factors that control temperature. Read on.