Prioritize...
This section is about tornadoes and the storms that most typically produce them -- supercells. By the end of this section, you should be able to define tornadoes and funnel clouds, describe the tornado climatology in the United States (including geographic locations and monthly distribution), and describe where the rotation of a supercell and tornado comes from.
Read...
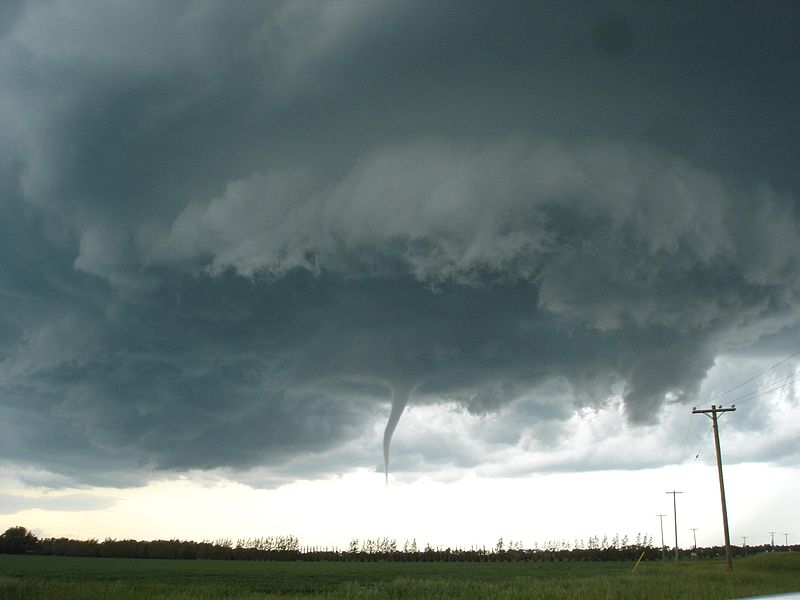
While there's no doubt that damaging straight-line winds are a major thunderstorm hazard, tornadoes tend to make the big headlines. Although I've described tornadoes before (and you're probably somewhat familiar with them), formally a tornado is a rapidly rotating column of air in contact with the ground and with the base of a cloud. Note that, by definition, a tornado must be in contact with the ground. There's no such thing as a tornado that's not on the ground, so if you've ever seen storm-chaser video of a tornado and someone yelled "Tornado on the ground!" they were being redundant. If there's a rapidly rotating column of air that's descending from a cloud, but it doesn't touch the ground, it often appears as a funnel cloud (see the photo on the right for an example).
Tornadoes can vary in shape and size, but the average tornado is only a few hundred feet wide. On the large end of the spectrum, tornadoes can exceed two miles in width, so in the scheme of things, tornadoes are pretty small weather features, but they can be devastating. Even so-called "weak" tornadoes have wind gusts around 65 miles per hour or more (plenty strong enough to damage property). On the other hand, the strongest tornadoes can attain wind speeds of more than 200 miles per hour, which can flatten entire neighborhoods and grab top headlines.
As you may recall, most tornadoes (and nearly all strong tornadoes) are caused by supercell thunderstorms (thunderstorms characterized by a rotating updraft), so tornadoes can happen anywhere that supercell thunderstorms can develop. Statistics show that the United States is the world leader in tornadoes (averaging more than 1,000 per year), followed by Canada (a distant second at roughly 100 per year). Several other regions of the world regularly experience tornadoes, however (shaded in orange on this world map from the National Climatic Data Center).
Within the United States, tornadoes have occurred in all 50 states, although they're much more common in some states compared to others. The map below shows the average annual number of tornadoes per 10,000 square miles in each state from 1995 to 2014. First, take note that the states in the western U.S. labeled with zeroes merely average less than one tornado per 10,000 square miles per year (so tornadoes are infrequent, but not unprecedented). Secondly, the Great Plains, Midwest, and Southeast U.S. tend to be "hotspots" for tornadoes (states like Kansas, Oklahoma, Iowa, Illinois, Mississippi, Alabama, and Florida). Alaska and Hawaii aren't shown on the map, but tornadoes occur in those states, too (although infrequently, especially in Alaska).
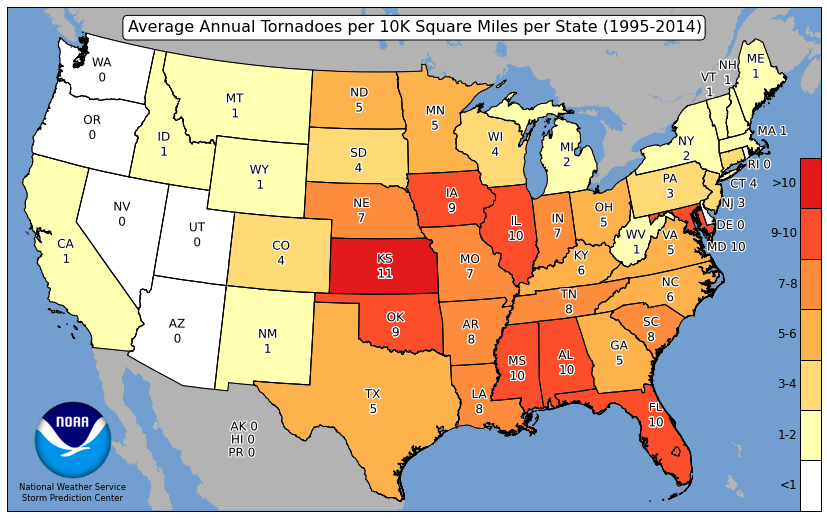
The relative frequent occurrence of tornadoes in much of the Great Plains compared to other parts of the country has earned it the nickname "Tornado Alley." But, given the frequency of tornadoes in the Southeast, forecasters are starting to recognize a secondary "tornado "alley" that extends across much of the Southeast U.S., sometimes referred to as "Dixie Alley." Why are these zones so ripe for tornadoes? Well, for starters, they most frequently possess the ingredients for supercell thunderstorms. You may recall that supercell thunderstorms require instability for positively buoyant air parcels and strong vertical wind shear. But, the formation of individual supercells also requires weak or modest sources of lifting (remember that when lifting is really strong, lots of thunderstorms form in close proximity, and squall lines often develop). In short, favorable ingredients for supercells and tornadoes come together in "Tornado Alley" and "Dixie Alley" more often than in other parts of the country.
Throughout the year, tornado activity also has a seasonal "rhythm" to it, as this animation showing the probability of a tornado on select dates throughout the year shows. The lifting that comes along with mid-latitude low-pressure systems along with access to maritime-Tropical air can be a volatile recipe for severe thunderstorms and tornadoes, and those ingredients typically come together most favorably along the Gulf States in the winter. As winter turns to spring, the likelihood of tornadoes increases farther north into the Great Plains, as the northward extent of maritime-Tropical air increases. The extent of tornadoes in the summer months spreads even farther north, and even toward the Northeast U.S., when warm, buoyant air is more readily available. Late in the year as summer turns to fall and winter, the likelihood of tornadoes decreases again (and migrates southward again). In terms of overall numbers, April, May, and June are typically the most active months for tornadoes, with the peak of tornado activity occurring in May, on average (credit: ustornadoes.com).
Putting a Spin on Supercells
So, why are supercells the most common producers of tornadoes? It all comes down to spin. Supercells are characterized by a sustained, rotating updraft, but what causes the rotation? When I introduced supercells, I discussed the importance of strong vertical wind shear in increasing the storm's longevity by separating the storm's updraft and downdraft, which ensures that the downdraft doesn't interfere with the updraft. But, vertical wind shear is important for inducing rotation into the storm, as well. In particular, it's wind shear in the lower troposphere that's critical.
To use an analogy, I like to think of supercells as getting their spin by "eating" long, spinning "spaghetti noodles" of warm, moist air. How does a supercell "eat" spinning spaghetti noodles? When there's strong vertical wind shear in the lower troposphere, wind speeds increase markedly with increasing altitude, which would impart spin on a horizontal "noodle" of air aligned with the flow into the storm's updraft. If you're having trouble visualizing, take (or imagine) a pencil resting on the palm of your left hand and then move your right palm over the pencil to simulate faster winds above -- the pencil should roll across your hand, which is similar to the process of how faster winds above the surface can produce a horizontal "noodle" of rotating air.
As the twirling "noodle" of air gets drawn into the storm's updraft, the rotating air gets tilted vertically, resulting in the formation of a mesocyclone, which rotates around a vertical axis. The annotated photograph below should help you visualize a long, spinning "spaghetti noodle" getting ingested into a supercell's updraft. Or, if a spaghetti noodle visualization doesn't do it for you, think of a stream of perfectly spiraling footballs flowing toward the storm and then tilting vertically as they get sucked into the updraft.
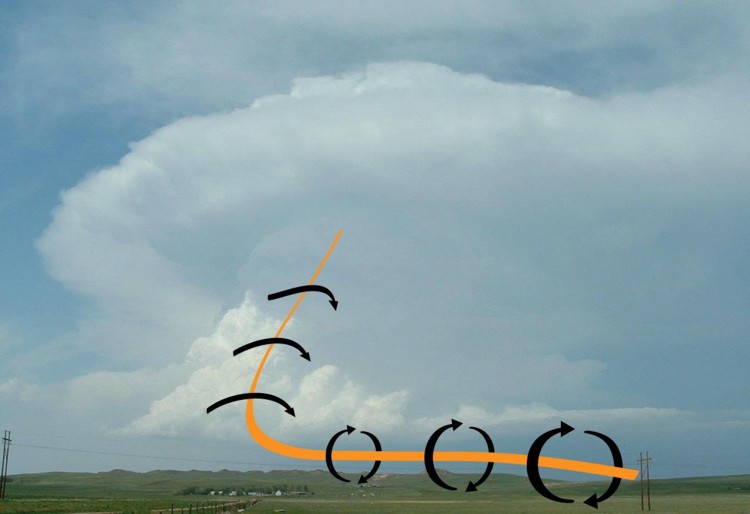
But, the presence of a mesocyclone doesn't guarantee that a tornado will form. The spinning column of air that is the mesocyclone must get further "stretched" vertically, which causes the column to spin more rapidly, via the conservation of angular momentum. If you've ever watched a figure skater perform a scratch spin, you've seen this concept in action. As the skater pulls her arms in and raises them above her head, she spins faster as a result of the conservation of angular momentum. By pulling her arms in, she decreases her radius of rotation, and increases her spin rate. This process is a critical part of the transition from a mesocyclone to a tornado -- the updraft must contract (become "skinnier") and stretch vertically in order to spin faster, paving the way for a tornado.
How and why does this vertical stretching occur? Why does it happen in some supercells and not others? Good questions! Unfortunately, they're questions that we don't have concrete answers for currently. Some emerging theories suggest that the buoyancy of air parcels and the relative humidity of air parcels in the lower troposphere are important factors. If they're not "just right," a tornado won't form. We ultimately don't know the exact details of the mechanisms that form tornadoes yet, but meteorologists are still actively studying the process through observations taken during field experiments. Measuring the environment in and immediately surrounding a tornado has historically not been easy! In fact, if you've ever seen the movie 1996 movie, Twister, the chasers' devised method for measuring a tornado's environment was actually based on a real research project from the 1980s.
So, that's the story of where and when tornadoes most commonly form, and how a supercell gets the spin that can produce a tornado. Up next, we'll explore the anatomy of a supercell more closely.