Insolation
Insolation — Incoming Solar Radiation
It all starts with the Sun, where the fusion of hydrogen creates an immense amount of energy, heating the surface to around 6000°K; the Sun then radiates energy outwards in the form of ultraviolet and visible light, with a bit in the near-infrared part of the spectrum. By the time this energy gets out to the Earth, its intensity has dropped to a value of about 1370 W/m2 —as we just saw this is often called the solar constant (even though it is not truly constant — it changes on several timescales):
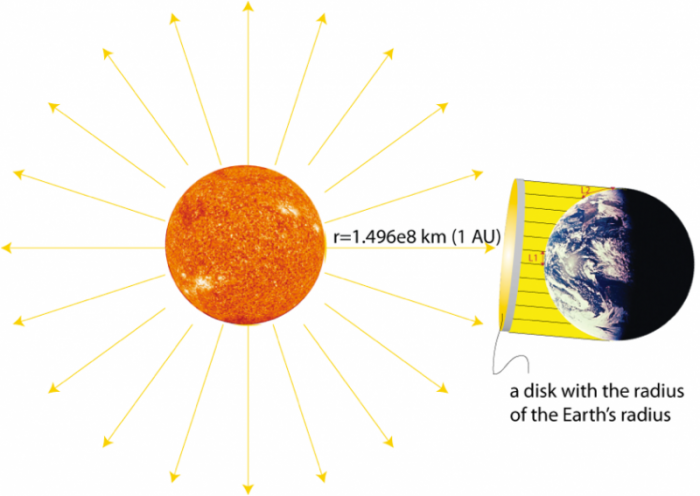
Since the Earth spins, the insolation is spread out over an area 4 times greater than the disk shown in the figure above, so the solar constant translates into a value of 343 W/m2. This is a bit less than six 60 Watt light bulbs shining on every square meter of the surface, which adds up to a lot of light bulbs since the total surface area of Earth is 5.1e14 m2. How much energy do we get from the Sun in a year? Take 1370 W/m2, multiply by the area of the disk (pi x r2 where r=radius of Earth, 6.37e6 m), and this gives us an answer in Watts, which has units of joules per second, so if we then multiply by the number of seconds in a year, then we get the total energy in joules per year. The number is staggering — 5.56e24 Joules of energy — and is 10,000 times greater than all of the energy generated and consumed by humans each year.
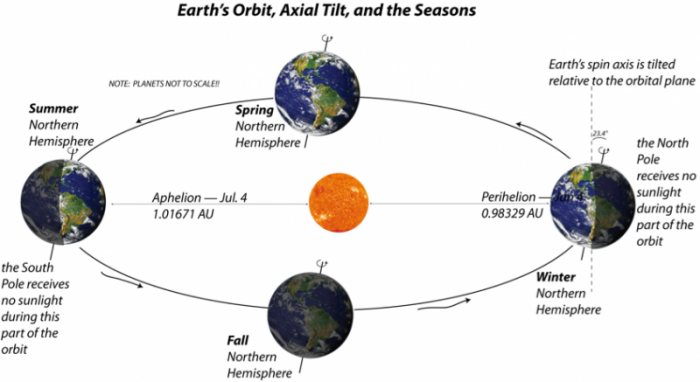
The insolation is not constant over the surface of the Earth — it is concentrated near the equator (first figure on the page) because of the curvature of the Earth. But, the situation is complicated by the fact that the Earth’s spin axis is tilted by 23.4° relative to a line perpendicular to the Earth’s orbital plane (see the second figure on the page), so that as Earth orbits around the Sun, the insolation is concentrated in the Northern Hemisphere (the Northern Hemisphere summer) and then the Southern Hemisphere (winter in the Northern Hemisphere). This tilt of the spin axis, also called the obliquity, is the main reason we have seasons.
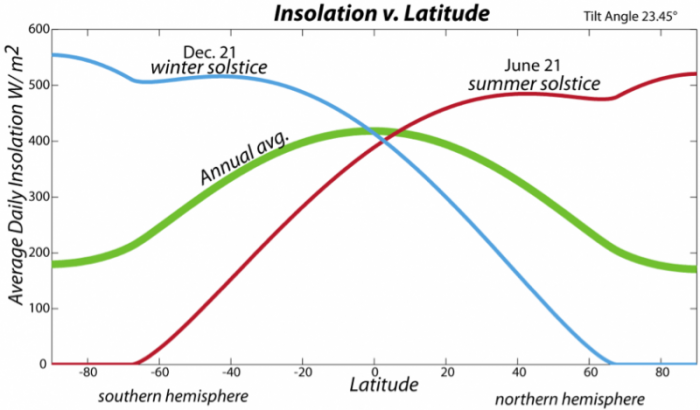
The tilt of the spin axis also means that day length changes, and these changes are most dramatic at the poles, which experience 24 hours of daylight during their summers and no daylight during their winters. The varying day length, along with the angle of incidence of the Sun’s rays, combine to control the average daily insolation variation (see figure above). On a yearly average, the equatorial region receives the most insolation, so we expect it to be the warmest, and indeed it is.
Earlier, we mentioned the Solar Constant — a measure of the amount of solar energy reaching Earth. In reality, this value is not a constant because the Sun is a dynamic star with lots of interesting changes occurring. One of the best known of these changes is the solar cycle, related to sunspots. Sunspots are dark regions on the surface of the Sun related to intense magnetic activity, and measurements have shown that the greater the number of sunspots, the greater the energy output of the Sun. Early observations of these sunspots revealed a pronounced cyclical pattern to them, varying on an 11-year cycle, as shown below.
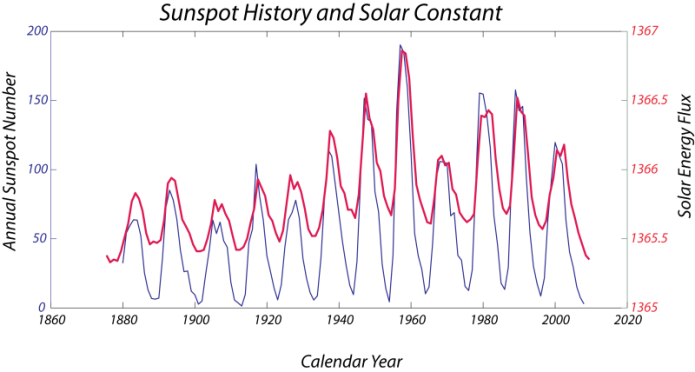
Here, in blue, we see the annual number of sunspots and in red we see the reconstructed solar intensity or Solar Constant. The reconstruction is made by studying the relationship between sunspot number and solar intensity in the last few decades, where we have good direct measurements of the solar intensity — this provides a relationship that is fairly simple and directly proportional. Higher sunspot numbers correspond to higher solar intensity. Both records are characterized by a strong 11-year cycle, often called the sunspot cycle.
The magnitude of variation in the Solar Constant, however, is quite small, and we shall see in our lab activity for this module that this amounts to a very small change in the temperature of the Earth.