Ocean Acidification
Background
Increasing levels of CO2 in the atmosphere are slowly causing the surface of the ocean to become more acidic. This is because the ocean absorbs some of the CO2, forming a weak carbonic acid. At present, the ocean absorbs about a third of fossil fuel emissions, but this amount is likely to increase to 90% in the future. Over the last century, the average pH of the ocean has decreased, and there are hints that the current levels are beginning to impact organisms that make their shells out of the minerals aragonite and calcite (both composed of CaCO3). Aragonite is more susceptible to dissolution in more acidifc waters than calcite. Coral reefs that are made of the mineral aragonite and are particularly vulnerable to ocean acidification. A recent study has found, for example, that the area of coral covering the Great Barrier Reef in Australia has been cut in half since 1985. However, coccolithophores and foraminifera, organisms that serve a vital role at the base of the marine food chain that are composed of calcite, are becoming increasingly susceptible. Moreover, the future appears to be even more bleak; some CO2 projections suggest by the year 2100 there will be a 150% increase in the ocean’s acidity compared to preindustrial times. Here we review the chemical changes in seawater that result from increasing CO2, and then we discuss the impact on reefs and planktonic organisms in the ocean. Finally, we discuss the evidence for acidification in ancient oceans and its impact on life in the past.
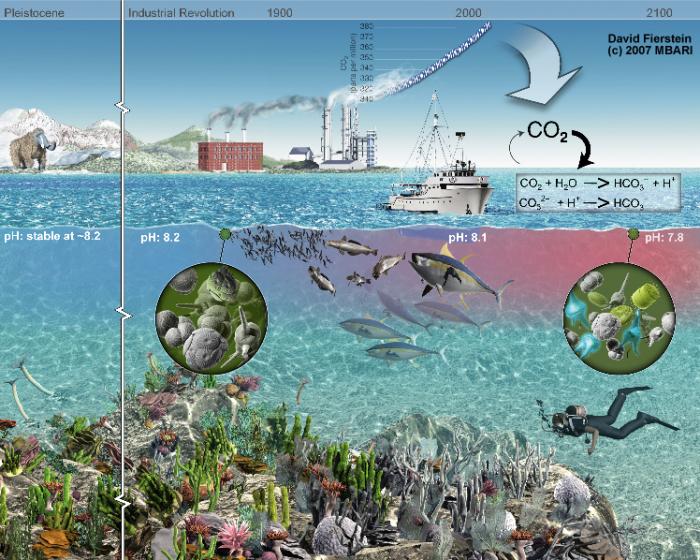
The following video provides a thorough overview of the potential impact of acidification on the oceans.
Video: Acid Test: The Global Challenge of Ocean Acidification (21:34)
[MUSIC PLAYING]
SIGOURNEY WEAVER: The ocean keeps secrets. Hidden beneath its waves are worlds within worlds. Over billions of years, the ocean has created endless varieties of life, life that enchants us, that sustains us. And despite our science, life that mystifies us still.
STEVE PALUMBI: The oceans are an incredible place full of the most amazing kinds of life, life that you can never imagine really working. Things that if somebody just thought of them and showed them to you, you'd think, that's ridiculous. Nothing like that could ever live. But it does.
BRUCE STEELE: In 35 years of diving, it's quite a picture. I've spent my life on the bottom of the ocean with black sea bass. I've seen white sharks underwater. I've been in schools of bait that would be so big that they will dark out the sun. I can only hope that the ocean maintains that vitality. It's an incredible place of mystery, and it's something that's beautiful beyond description.
LISA SUATONI: People rely on the oceans in so many ways. Some ways are obvious, like food, recreation, transportation.
STEVE PALUMBI: They clean our shores. They protect our coastlines from storms.
LISA SUATONI: The oceans regulate climate and provide the world with most of its oxygen.
SIGOURNEY WEAVER: But we are now certain of one awesome fact. The ocean's power to create life is rivaled by our own power to destroy it.
[MUSIC PLAYING]
Scientists refer to ocean acidification as the other carbon problem. The first, of course, is global warming.
LISA SUATONI: People have heard about global warming for years, but it's only over the past five years that experts really understood that the carbon dioxide is causing a problem for the oceans as well. And what's worrisome is it hasn't even been on our radar.
SIGOURNEY WEAVER: Carbon dioxide pollution is transforming the chemistry of the ocean, rapidly making the water more acidic. In decades, rising ocean acidity may challenge life on a scale that has not occurred for tens of millions of years. So we confront an urgent choice, to move beyond fossil fuels or to risk turning the ocean into a sea of weeds.
KEN CALDEIRA: When we burn coal, oil, and gas, we introduce carbon dioxide into the atmosphere. But the atmosphere touches the ocean over 70% of Earth's surface. [THUNDER] So this carbon dioxide we're putting into the atmosphere we are also getting into the ocean.
SIGOURNEY WEAVER: Carbon dioxide, or CO2, exists naturally in our atmosphere. Plants need it to grow. Animals exhale it in every breath. But carbon dioxide is also a byproduct of burning fossil fuels. And in large amounts, it is a dangerous pollutant. Since the Industrial Revolution, the ocean has absorbed roughly one-quarter of the carbon dioxide produced by burning fuels. Scientists once thought this beneficial. After all, that carbon dioxide would otherwise accelerate global warming. But what happens when so much carbon dioxide, 22 million tons of it each day, mixes with ocean water? In terms of chemistry, the answer is simple. It becomes an acid.
LISA SUATONI: Since the Industrial Revolution, the ocean acidity has increased by 30%. With mathematical models, scientists have demonstrated that if we continue to pollute as we are now, the ocean acidity will double by the end of the century compared to pre-industrial times. That is a big problem.
SIGOURNEY WEAVER: Scientists only recently stopped to think about what this would mean for life in the ocean. Thousands of ocean species build protective shells to survive. Some are so prolific they can be seen from space. These organisms create their shells, which can be paper thin, by drawing certain molecules from the water around them. But rising acidity depletes those molecules.
KEN CALDEIRA: So by removing the essential building block for shell formation, it's making the organisms work a lot harder to build their shells. And that means they have less energy to get food. They have less energy to reproduce. And eventually, the organism can no longer compete ecologically. The surprise is how sensitive some marine organisms are to this increased acidity from carbon dioxide.
SIGOURNEY WEAVER: And when acidity gets too high, shells dissolve.
STEVE PALUMBI: We're changing the basic rules of everything. And because of that, a lot of organisms may not be able to survive.
KEN CALDEIRA: Already we've seen water showing up off the coast of northern California that's acidic enough to start actually dissolving sea shells. It's thought that this kind of corrosive water showing up will become more and more common.
BRUCE STEELE: Most of the West Coast of North America's shellfish-- that's Dungeness crabs, lobsters, mussels, oysters, sea urchins, shrimp-- all those life forms are at risk.
KEN CALDEIRA: By mid-century, if we continue emitting carbon dioxide the way we have been, entire vast areas of both the Southern Ocean and the Arctic Ocean will be so corrosive that it will cause seashells to dissolve.
SIGOURNEY WEAVER: Scientific models show that in just a few decades we will profoundly alter the ocean's chemistry. Such conditions haven't existed since the extinction of the dinosaurs. Recreating those conditions so quickly could leave many ocean animals unable to adapt. What if shellfish could no longer build shells? Would they cease to exist? Perhaps. Shelled creatures such as corals and plankton play a key role in the ocean food web. Terrapods are a kind of plankton that live all around the world and in great abundance in polar waters. Terrapods are especially vulnerable.
SPEAKER 6: Should I focus in that?
VICTORIA FABRY: Yeah, maybe right in here.
SPEAKER 6: Right in there?
VICTORIA FABRY: Mm-hm. We're looking at terrapod shells, which are planktonic snails with a calcium carbonate shell, that we collected from Antarctica this past winter. And you can see it looks like there's this lip where it may have already started to dissolve and kind of curled over. Because that's what it looks like when it dissolves. It kind of melts, almost like a candle and wax melting. The shell thickness along that leading edge right here is less than one micrometer thick. These are the thinnest terrapod shells I've ever seen.
SIGOURNEY WEAVER: There's growing alarm that higher acidity will extinguish creatures like terrapods that are a basic food source for fish. In many parts of the world, fish are a basic food source for people.
STEVE PALUMBI: So you can't just worry about the big things in the ocean. You have to worry about what they eat and where their food comes from. If the smallest things in the ocean are affected by ocean acidification, then it ripples all the way up the food web, making the largest things in the ocean even more endangered.
[WHALE SONG]
SIGOURNEY WEAVER: As individual strands disappear, the entire food web becomes weaker, more vulnerable, less beneficial to humans.
OVE HOEGH-GULDBERG: And many of us are concerned about what that means for the Earth's marine ecosystems, but also for the many millions of people that depend on these systems for their food and income.
SIGOURNEY WEAVER: Ocean acidity will rise most quickly in cold water regions and areas where deep water wells up to the surface.
LISA SUATONI: That is disconcerting because it coincides with the regions of the most productive fisheries in the world.
BRUCE STEELE: I'm a fisherman. Every single day I have to make a prediction where I'm going to go fishing, whether I'm going to find fish where I go. And every single day the decisions I make make the difference between whether I stay a fisherman and make a profit. I can make predictions. I think these things are dire problems. Either we change what we're doing on land, or it will have profound effects on fisheries as we know them.
SIGOURNEY WEAVER: Marine life that might withstand warming temperatures or rising acidity may succumb when confronted by both. Coral reefs already struggle to survive in warming waters. Rising ocean acidity puts them in double jeopardy.
OVE HOEGH-GULDBERG: We know that coral reefs are particularly sensitive to ocean acidification. And the reason for that is that corals are unable to form their skeletons as quickly as they used to. And reefs are starting to crumble and disappear. We may lose those ecosystems within 20 or 30 years. And in those structures live an estimated million species. One in every four species in the ocean lives on a coral reef. We've got the last decade in which we can do something about this problem. But it's very, very clear that if we don't start to deal with it right now with very, very stern cuts to emissions, we are going to condemn oceans to an extremely uncertain future.
KEN CALDEIRA: We're really in the last decades of coral reefs on this planet for at least the next, say, a million-plus years unless we do something very soon to reduce CO2 emissions. We're moving from a world of rich biological diversity into essentially a world of weeds.
STEVE PALUMBI: Today we're in a really remarkable history of the ocean. 100 years ago it was inexhaustible. You couldn't touch it. You couldn't harm it. In 100 years, it might be dead.
KEN CALDEIRA: When people say there was high CO2 100 million years ago so we have nothing to worry about, that high CO2 was achieved over a slow process of millions of years. And if we achieve high CO2 over millions of years, the Earth will be able to handle it. If we achieve high CO2 over decades, the ocean is in big trouble.
SIGOURNEY WEAVER: Earth is the only planet we know of where life exists. To understand our own actions, we sometimes need to view them in a larger context. Planet Earth was formed 4 and 1/2 billion years ago. 3 and 1/2 billion years ago, life began. 250 million years ago, dinosaurs appeared. And 200,000 years ago, homo sapiens. Within that framework, human civilization is brand new, our industrial society but an instant. Yet in that instant, we have altered the course of nature. We have heated the Earth's surface, acidified its oceans, and consumed much of its natural habitat. Now something extraordinary looms, a mass extinction of animals and plants caused not by volcanic eruption or the collision of a meteor but by the actions of one species, ours.
KEN CALDEIRA: If we destroy these ecosystems, it will take millions of years for them to recover. It's as if somebody, just because they had the ability to do it, decided to run through the Metropolitan Museum with a knife slashing the great paintings of the world.
SIGOURNEY WEAVER: We have created this problem. We should be able to solve it. The ocean, after all, is resilient. Given the chance and enough time, it can heal itself. So how can we give the ocean that chance? Marine protected areas like national parks in the sea shelter ocean life from industry and development. Sustainable fishing practices allow fish stocks to regenerate. The ocean can better defend itself against rising acidity and temperature if its systems are healthy.
LISA SUATONI: To make the oceans more resilient to these changes, we need to do a better job of keeping the oceans healthy. That means restoring depleted fish populations, establishing marine protected areas all around the globe, and reducing pollution, particularly nutrient pollution in the coastal zones.
STEVE PALUMBI: Solving those local problems gives those ecosystems a chance to survive, a chance to make it through while we solve the global problem. We know how to solve the local problems of marine ecosystem health. We know how to solve the global problems. The question is, will we?
[JET ENGINE]
SIGOURNEY WEAVER: The only way to stop acidification is to emit less carbon dioxide. Our Industrial Revolution began more than two centuries ago. Technology has advanced rapidly since then. But we still make energy as we have for hundreds of thousands of years, by setting things on fire. Often, we squander the energy we make, using more than necessary to accomplish our goals. But now we know how to use energy more efficiently, how to do more with less.
RALPH CAVANAGH: There was a time when people thought about energy efficiency and conservation as sacrifice, doing without-- dark homes, shuttered economies. That is emphatically not what we're talking about. We're talking about getting dramatically more work out of less energy with better technology. Those energy efficiency solutions are particularly promising because the whole world will want to adopt them. If we take that initial step, we will also, in addition to reducing carbon pollution, have the very welcome dividend in the form of economic stimulus because we will be reducing energy bills.
SIGOURNEY WEAVER: We know how to capture energy cleanly from sunlight, wind, tides, and the heat of the Earth's core.
RALPH CAVANAGH: Imagine that you're living in a house that gets some of its electricity from its own solar panels, feeds some of that back into your own vehicle when it's plugged in at night, provides you with energy services-- and maybe this is the most important single piece of it-- at costs below those you're paying now. That double dividend was never more needed by the US and world economy than it is right now.
SIGOURNEY WEAVER: We are on the verge of a green industrial revolution, a revolution that will expand our economy, protect our resources, and give us real energy independence. There is much we don't know about how carbon pollution will affect our world. Still, we have to choose. We can go on as we have, forcing future generations to survive somehow without the vast ocean resources that have sustained us. Or we can move beyond fossil fuels, securing a future that works for all of us, for all living things. What will we choose?
[MUSIC PLAYING]
KEN CALDEIRA: You're not going to use this. I'm going to just say it, though. What makes a Greek tragedy a tragedy is that you can see it coming. Oedipus goes and marries his mother and eventually tears his eyes out. And you want to tell him, look, no, don't marry your mother. You can stop this process now.
STEVE PALUMBI: And you think, if I could only go back and change that one little tiny instant, then things would have been different. I think we're in that instant right now.
KEN CALDEIRA: We sit by feeling almost helpless because we see this unraveling, leading to its tragic end.
LISA SUATONI: Researchers are predicting significant substantial changes in the next two decades to our oceans. So it is not necessarily a problem we're passing off to future generations. It's a problem that we're generating for ourselves.
OVE HOEGH-GULDBERG: I think what gets me up in the morning is that I don't want to see coral reefs disappear on my watch. And I know that my fellow scientists feel this way as well. So we feel compelled to communicate the message that this is a serious issue and that changes that haven't happened for millions of years are starting to happen right before our eyes. I think it's important to point out that it's not all over yet.
BRUCE STEELE: I don't expect people to understand what terrapods or what various forms of plankton are likely to survive or not survive. But I do expect our policy-makers to take serious an issue that is so closely tied to life on this planet and the future of life on this planet. I have hope. You can't fish and not have hope.
Chemistry 101
The ocean contains a massive reservoir of dissolved CO2, hundreds of times more than in the atmosphere, and, actually, by contrast, the amount derived from fossil fuel burning is relatively modest. Since the beginning of the industrial revolution, about 340 to 420 petagrams carbon (a petagram or Pg is 1015 grams) in the form of CO2 has been emitted to the atmosphere, with about a third of that amount absorbed by the ocean, approximately 118 Pg. Seawater today may already contain more CO2 than at any time in many millions of years.
As we discussed in Module 5 on the Carbon Cycle, the absorption of CO2 in the ocean forms weak carbonic acid (H2CO3). Some of this acid dissociates in seawater releasing H+ ions, which make the water more acidic, as well as HCO3- (bicarbonate ions) and CO32- (carbonate ions). This reaction is as follows:
CO2(aq) + H2O = H2CO3 = H+ + HCO3- = 2 H+ + CO32-
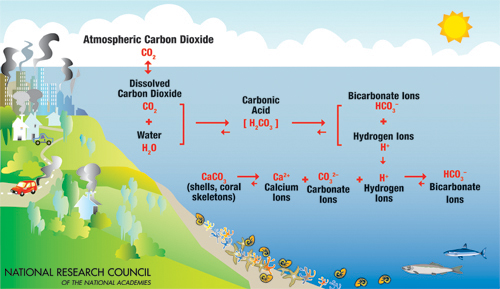
Going back to your elementary chemistry course, you might remember that a pH of greater than 7 is regarded as alkaline whereas a pH of less than 7 is acidic. Surface ocean waters have a pH of between about 7.9 and 8.3, which means that they are, by definition, alkaline. Anthropogenic CO2 is thought to have decreased the mean pH of the ocean by 0.1 unit since 1800. This may not sound like that much, but more ominous is the projection that if CO2 levels continue to rise unabated (i.e., projections based on SRES A2 “business as usual”, pH levels will drop a further 0.3 by 2100. As we will see below, in parts of the ocean, these levels would be extremely damaging to organisms that build their skeletons out of CaCO3, which is very sensitive to CO2 addition.
CaCO3 is the dominant material used by invertebrate organisms to build their skeletons. There are two different minerals made of CaCO3, known as polymorphs: calcite and aragonite. These minerals have the same composition but different crystal lattice structure and thus their properties and behavior in seawater differ, including their ability to dissolve. To understand how CaCO3 dissolves and precipitates, we need to introduce a term Ω that represents the saturation state of the water. Where waters are highly saturated with respect to CaCO3 and Ω is high, calcite and aragonite are less likely to dissolve than where these waters are less saturated or even undersaturated and Ω is low. Likewise, calcite and aragonite are more likely to precipitate under higher Ω values. The dissolution and precipitation reactions are as follows:
Dissolution reaction: CaCO3 (solid) = Ca2+ + CO32-
Precipitation reaction: Ca2+ + CO32- = CaCO3 (solid)
An increase in CO2 from the atmosphere presents a double whammy for skeletons formed from CaCO3, both aragonite and calcite. The H+ ions and carbonate ions (CO32-) that derive from the dissociation of carbonic acid combine to form bicarbonate ions (HCO3-). This rapid reduction in available carbonate ions decreases Ω and limits calcification by organisms with aragonite- and calcite-based skeletons. However, here we need to dispel two myths. The first myth is that the precipitation of CaCO3 is directly controlled by pH. In fact, precipitation is affected principally by the decrease in CO32, which is coincident with the addition of H+ ions, and reduction in pH. The second myth is that precipitation of CaCO3 can occur in any water that is oversaturated with respect to the particular CaCO3 mineral. In fact, both corals and coccolithophores have been shown to have difficulty calcifying in environments when waters were actually oversaturated. Different organisms can calcify at very different Ω values, but for most the decrease in saturation that results from decreasing CO32- content is a direct threat to calcification. Fiinally, it is key to move that aragonite is more susceptible to dissolution than calcite. Thus, shells made of the CaCO3 polymorph aragonite, including the corals, will be the first to dissolve, followed by those made of the polymorph calcite.
The following video explains the threat of ocean acidification to the calcareous plankton.
Video: The Other Carbon Dioxide Problem (3:57)
Marine pteropods, or sea butterflies, are found throughout the ocean and are a major food source for fish and other organisms. In laboratory experiments, this pteropod shell dissolved over the course of 45 days, in seawater adjusted to an ocean chemistry projected for the year 2100.
What changes in the ocean might be putting pteropods at risk? The burning of fossil fuels has caused levels of carbon dioxide in the atmosphere to rise. This carbon dioxide, or CO2, doesn't just stay in the atmosphere. Roughly 30% of it is absorbed by the ocean. When co2 reacts with water, it forms carbonic acid, which quickly releases a hydrogen ion, or H+. The more hydrogen ions, or the lower the pH, or more acidic, a solution is. As CO2 levels increase over time, seawater will progress towards the acidic end of the pH scale, a process called ocean acidification.
We can make projections of this change in the ocean pH over time. It is expected that later this century, if CO2 emissions continue at the current rate, the average pH of the ocean will drop from 8.2 to 7.8. This relatively small decrease in pH may not seem significant, but it could impact many species of marine life. For pteropods, corals, and other species that depend on shells and exoskeletons, ocean acidification will lead to a decreased availability of dissolved calcium and carbonate, the chemical building blocks they use to make their shells and skeletons. If ocean chemistry changes as expected, shells with calcium carbonate mineral structures may begin to dissolve, depending on where they live in the ocean.
How do we know that ocean acidification is happening? Scientists at NOAA, and across the world, have measured these chemical changes over the past 40 years during research cruises, taking hundreds of thousands of ocean water samples. Shown here are the results from cruises in 1991 and 2005, where the availability of the shell mineral was sampled. In both years the highest concentration of available shell minerals were found in the surface waters, as shown by the dark yellow colors. Deeper waters naturally have very low availability of these minerals. However, when we look at how these values have changed between 1991 and 2005, we see that the availability of the shell mineral decreased much more in the surface waters, as indicated by the blue colors. In other words, ocean acidification is impacting shallower areas much more than deep areas. Shallow waters are where the bulk of the ocean productivity occurs, including the most diverse and economically important species and habitats. And these changes aren't occurring in just certain parts of the ocean, they're occurring throughout the entire ocean.
Today, repeated research cruises and permanent sampling stations continue to monitor changes in pH and availability of calcium carbonate minerals. As the ocean approaches a critical transition between shell building and shell dissolving, food webs in the world's oceans could be impacted. Phytoplankton and zooplankton, like pteropods, form the basis of most oceanic food chains. Coral reefs form the foundations of the most diverse marine habitats. And shellfish, such as oysters and crabs, and finfish, such as salmon, support economically important fisheries in many of the world's coastal communities. People depend on the viability of these species to ensure a healthy future of our own.
The saturation of CaCO3 in the oceans is also a function of temperature and pressure. A delicate balance exists between the production of CaCO3 via the formation of skeletons in the shallow part of the ocean and the dissolution of this aragonite and calcite in the colder and deeper realms of the ocean where waters are less saturated. In most parts of the ocean, undersaturation occurs far below the surface. However, recent increase in dissolved CO2 is leading to a shoaling of the saturation horizon of CaCO3, and, in the future, this will impact especially the organisms that live at depth or in colder waters as well as those that make their shells of the mineral aragonite, which is more soluble in seawater than calcite.